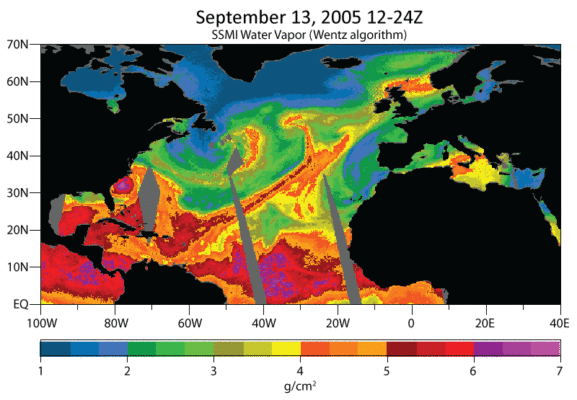
Extreme precipitation events, i.e., heavy rain episodes of short duration, can lead to severe or even catastrophic social and economic impacts, as seen recently in different flooding and landslide incidents throughout the world. One of the drivers behind these events is the occurrence of atmospheric rivers (ARs), a mechanism that transports great amounts of water vapour across the globe, and significantly controls the volume of precipitable water (rain or snow) once it reaches land. ARs are shallow (1–2.5 km in height) and narrow (∼300–500 km in width) plumes with high water vapour content, stretching over distances of at least 2,000 km. These structures are responsible for at least 90% of the atmospheric vertically integrated water vapour flux from the subtropics to the mid-latitudes. They are often associated with the pre-cold-frontal region of extratropical cyclones in the region of the warm conveyor belt and with strong winds at low levels of the atmosphere [1].
ARs play a major role in regulating the water cycle in many coastal regions [2], and are therefore fundamental for water resources management, an issue that is already taking the spotlight as a worldwide concern due to climate change. Hydrometeorological extremes can have opposite scenarios – more frequent or less frequent precipitation events – which either case can have severe socioeconomical impacts, such as flooding or severe droughts at particular regions. That is why it is fundamental to study the origin, development, and landfall mechanisms of the ARs, either from a climatological point of view, towards improving the management of the water supply either in the present but also in future scenario.
History and characterization
The term “atmospheric river” was coined in the late 90’s around the time remote sensing allowed for the measuring of vertically integrated, total column water vapour, although “tropospheric river” had appeared previously [3]. Over the last two decades, works on the subject multiplied, particularly when their association with flood events was discovered. Their impacts on the North American west coast were addressed from the late 20th century on, whereas in Western Europe and other regions studies began to proliferate since the late 2000s when it became clear that ARs were also strongly associated with water supply and hydroclimate worldwide.
Climatologically, there are more ARs during winter because they are typically associated with extra-tropical cyclones, which are prevalent in winter. The region of the world with the highest occurrence of ARs is the northeaster Pacific [4]. Across western Europe and the west coast of the U.S.A. ARs can bring up to 50% of the monthly rainfall [5].
Overall, there are two main mechanisms of AR landfall: coastal orographic features and storm-derived dynamics. When an AR encounters mountainous terrain, water vapour is forced upwards, which leads to orographic enhancement of rainfall, the key factor for extreme precipitation events and consequent flooding. For instance, this is the prevailing mechanism associated with the AR precipitation in the northeast Pacific. Other processes that can influence the intensity and duration of precipitation events, are convective vertical air motions and mesoscale frontal waves, typical of western Europe.
The importance of studying Atmospheric Rivers
Understanding these structures and their dynamics is crucial to improve the ability of forecasting, and from there to act towards preventing or mitigating their consequences. Identifying and predicting an AR is possible if able to identify and predict the key characteristics of ARs, such as position, water vapour transport, and extent. In the case of ARs associated with storm systems, it is also relevant to assess wind intensity and duration, which will have consequences in the amount of precipitation produced and whether it will affect a given area for several days or if it passes by quickly.
The most striking and relevant application of ARs studying is the attempt to forecast or mitigate the impacts of extreme hydrological events related to their occurrence, that can mean property damage, heavy economical losses, and mortality. Extreme flooding episodes in mid-latitude regions have increasingly been documented and revealed to be associated with ARs landfall. The ten largest winter flood events in the UK from 1970 to 2009 were associated with the occurrence of an AR. ARs were found to be the primary drivers of flood damages in the western United States for the last 40 years, which increase exponentially with AR intensity and duration [6]. Extreme precipitation events can be of particular severity in urban environments, such as the event of November 1983 in southwestern Europe that produced flash flooding, urban inundations, and landslides in Lisbon (Portugal), causing considerable infrastructure damage and human fatalities. In December 2019, three consecutive extra-tropical storms stroke the Iberian Peninsula, two of them associated with ARs, and caused flash floods and landslides, leading to major infrastructure damage and casualties, although in the aftermath the conspicuous heavy rainfall meant an attenuation of the drought that was striking that region.
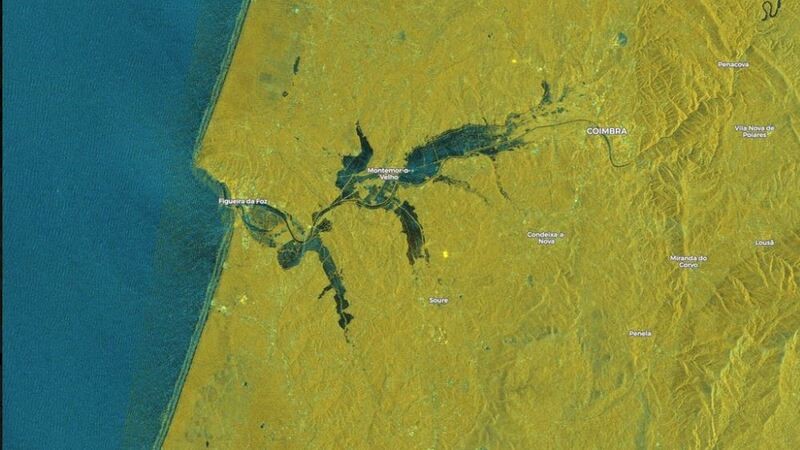
Figure 2. Large flooded areas in west Portugal following heavy rains due to the passage of three consecutive ARs in December 2019. Image from Sentinel1 of the European Space Agency (ESA). ©CopernicusEMS/Twitter.
While ARs and their associated extreme amount of vapor are relatively rare events over the polar regions, they have a significant impact on the precipitation climatology. For example, ARs are responsible for at least 10% of total accumulated snowfall across East Antarctica (localized areas reaching 20%) and a majority of extreme precipitation events [7]. Recent studies reveal that ARs may lead to increased snowfall and recovery of the ice shelves, as has occurred across Western Antarctica, or the increasing thawing in some parts of Greenland [8, 9].
What the future holds
For each 1ºC of temperature rise, the atmosphere can hold an extra 7% of water vapour. This means that, in a warming climate, atmospheric water vapour content will increase. On the other hand, the water vapour transport processes may also change. Overall, the studies point to stronger and more frequent ARs [10], which means that not only will the associated extreme events (floods, droughts) increase in intensity and frequency, but also that the hydrological balance at the regional scale may be at stake. However, due to their dependency on both weather and climate-scale processes, projections are uncertain. Addressing the multiscale nature of ARs and improving field data will be crucial for increasing the confidence of results of future studies.
Given also the impacts that ARs are increasingly found to have on ice and snow content, changes in their intensity and frequency, as well as the time of year they occur, will have consequences in the polar ice masses, either contributing to replenishing or to further loss of the ice sheets.
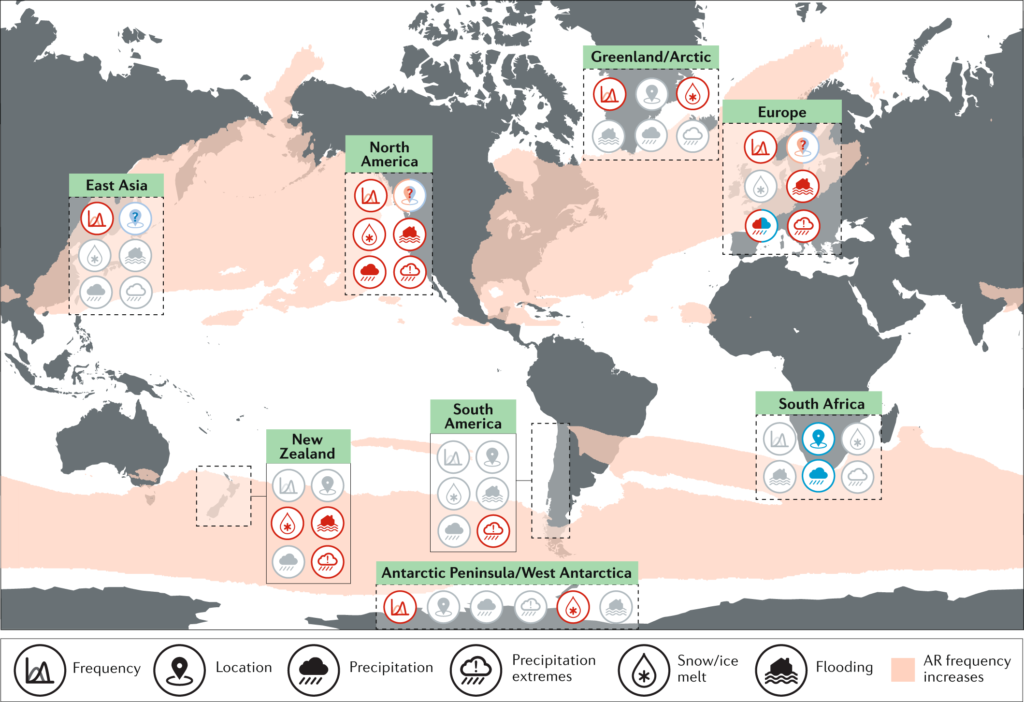
Figure 3. Projected changes and impacts in atmospheric rivers. Red and blue symbols reveal increases and decreases, respectively. Light red and blue symbols with ‘?’ indicate uncertainty in the projection. Grey symbols indicate unknown changes. Background shading illustrating AR frequency increases. Source: [10].
The IPCC 6th Assessment Report (Working Group I) summarizes these findings worldwide with regional differences [11]. Research in the past few years allows to conclude with high confidence that precipitation associated with extratropical storms and ARs will increase in the future in most regions, and to a lesser degree that poleward shift of storm tracks in the Southern Hemisphere and the North Pacific will bring about shifts in annual/seasonal precipitation. Conversely, precipitation not related to ARs is expected to decrease, which means in semi-arid regions the contribution of ARs to annual total precipitation will grow, from whence water supply issues will also be more dependent on extreme precipitation events. Intensification of short-duration intense rainfall can increase the severity and frequency of flash flooding. Flood and landslide hazard will increase worldwide, although their impacts depend on runoff conditions, soil use, soil moisture content, and snowmelt in mountainous regions. It is clear that these rivers in the sky are a major player in the Earth’s global climate system, either as a water source for a hydrological balance, thus preventing regional water scarcity and droughts, or as the origin of the proverbial “cats and dogs” downpouring, making mischief at the local level. With increasing temperatures and hydrological imbalances on the side of nature, accompanied by denser populations and less responsible land use and territory management on the side of mankind, episodes of flooding and landslides as consequence of AR occurrence are likely to become more frequent and severe. However, the scientific and technological tools available nowadays for forecasting and early warnings, seasoned with the need of clear communication between local authorities and the general public, are unprecedent as to give us the ability to adapt ahead of these events and to avoid as much as possible the loss of lives and material damages.
This blog was edited by the editorial board.
References: [1] Ralph, F.M., Dettinger, M.D., Cairns, M.M., Galarneau, T.J., and Eylander, J. (2018) Defining “Atmospheric River”: How the Glossary of Meteorology Helped Resolve a Debate, Bull. Am. Meteorol. Soc., 99, 4, 837–839, https://doi.org/10.1175/BAMS-D-17-0157.1. [2] Ralph, F.M., and Dettinger, M.D. (2011) Storms, floods, and the science of atmospheric rivers, Eos, 92, 265–266, http://doi.org/10.1029/2011EO320001. [3] Newell, R. E., Newell, N. E., Zhu, Y., & Scott, C. (1992). Tropospheric rivers?–A pilot study. Geophysical research letters, 19(24), 2401-2404. https://doi.org/10.1029/92GL02916 [4] Waliser, D.E., Moncrieff, M.W., Burridge, D., Fink, A.H., Gochis, D., Goswami, B.N., Guan, B., Harr, P., Heming, J., Hsu, H., Jakob, C., Janiga, M., Johnson, R., Jones, S., Knippertz, P., Marengo, J., Nguyen, H., Pope, M., Serra, Y., Thorncroft, C., Wheeler, M., Wood, R., and Yuter, S. (2012) The year of tropical convection (May 2008–April 2010): climate variability and weather highlights, Bull. Amer. Meteor. Soc., 93, 1189–1218, http://doi.org/10.1175/2011BAMS3095.1. [5] Lavers, D.A., and Villarini, G (2015) The contribution of atmospheric rivers to precipitation in Europe and the United States, J. Hydrology, 522, 382–390, https://doi.org/10.1016/j.jhydrol.2014.12.010. [6] Corringham, T.W., Ralph, F.M., Gershunov, A., Cayan, D.R., and Talbot, C.A. (2019) Atmospheric rivers drive flood damages in the western United States, Sci. Advances, 5, 12, eaax4631, http://doi.org/10.1126/sciadv.aax4631. [7] Wille, J. D., Favier, V., Gorodetskaya, I. V., Agosta, C., Kittel, C., Beeman, J. C., Jourdain, N.C., Lenaerts, J.T.M., and Codron, F. (2021) Antarctic atmospheric river climatology and precipitation impacts. J. Geophys. Res. Atmospheres, 126, 8, e2020JD033788, https://doi.org/10.1029/2020JD033788. [8] Wille, J. D., Favier, V., Dufour, A., Gorodetskaya, I. V., Turner, J., Agosta, C., & Codron, F. (2019). West Antarctic surface melt triggered by atmospheric rivers. Nature Geoscience, 12(11), 911-916. https://doi.org/10.1038/s41561-019-0460-1 [9] Neff, W. (2018). Atmospheric rivers melt Greenland. Nature Climate Change, 8(10), 857-858. https://doi.org/10.1038/s41558-018-0297-4 [10] Payne, A.E., Demory, ME., Leung, L.R., Ramos, A.M., Shields, C.A., Rutz, J.J., Siler, N., Villarini, G., Hall, A., and Ralph, F.M. (2020). Responses and impacts of atmospheric rivers to climate change, Nat. Rev. Earth Environ., 1, 143–157, https://doi.org/10.1038/s43017-020-0030-5. [11] Douville, H., Raghavan, K., Renwick, J., Allan, R.P., Arias, P.A., Barlow, M., Cerezo-Mota, R., Cherchi, A., Gan, T.Y., Gergis, J., Jiang, D., Khan, A., Pokam Mba, W., Rosenfeld, D., Tierney, J., and Zolina, O. (2021) Water Cycle Changes. In: Climate Change 2021: The Physical Science Basis. Contribution of Working Group I to the Sixth Assessment Report of the Intergovernmental Panel on Climate Change [Masson-Delmotte, V., P. Zhai, A. Pirani, S. L. Connors, C. Péan, S. Berger, N. Caud, Y. Chen, L. Goldfarb, M. I. Gomis, M. Huang, K. Leitzell, E. Lonnoy, J.B.R. Matthews, T. K. Maycock, T. Waterfield, O. Yelekçi, R. Yu and B. Zhou (eds.)], Cambridge Univ. Press, In Press.