November 13th, 1985, is a date that is still etched in my memory. This was the day that the Colombian town of Armero was submerged beneath a catastrophic flood of volcanic rocks, mud and water; a lahar that had swept down from the summit of the volcano Nevado del Ruiz, erupting about 40 kilometres away. For days, terrible scenes of anguish and despair filled our television screens, as rescuers str ...[Read More]
Volcán Calbuco: what do we know so far?
Detailed assessments of what happened during the April 22-23 eruption of Calbuco, Chile, are now coming in from the agencies responsible for the scientific monitoring of the eruption (SERNAGEOMIN) and for the emergency response (ONEMI). The volcano is well monitored and accessible, and as a result there has been a great deal of high quality information, and imagery, made available very quickly. In ...[Read More]
Calbuco erupts. April 22, 2015.
Volcan Calbuco, which burst into eruption on April 22nd, is one of more than 74 active volcanoes in Southern Chile that are known to have erupted during the past 10,000 years. Unlike its photogenic neighbour, Osorno, Calbuco is a rather complex and rugged volcano whose eruptive record has posed quite a challenge for Chilean geologists to piece together. The little that we do know about Calbuco’s e ...[Read More]
The fate of volcanic ash in the environment
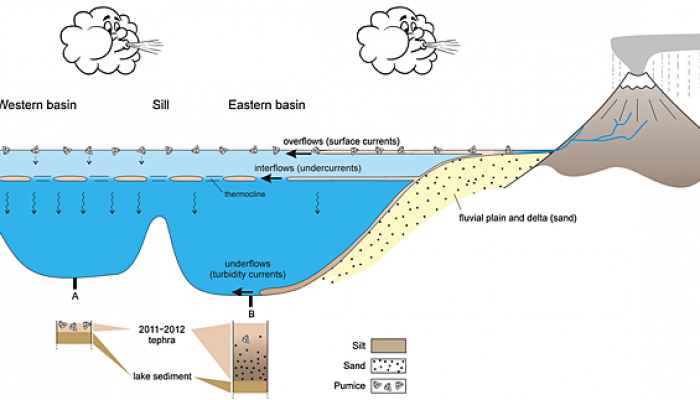
Over the past few years, we have been working to piece together the record of major post-glacial volcanic eruptions in southern Chile that have occurred over the past 18,000 years. This work started off with a search for volcanic ash layers that were preserved in road cuttings, or cliff faces other accessible geological locations in the region. Since then it has expanded to include the search for ...[Read More]
There’s (volcanic) dust in the archives
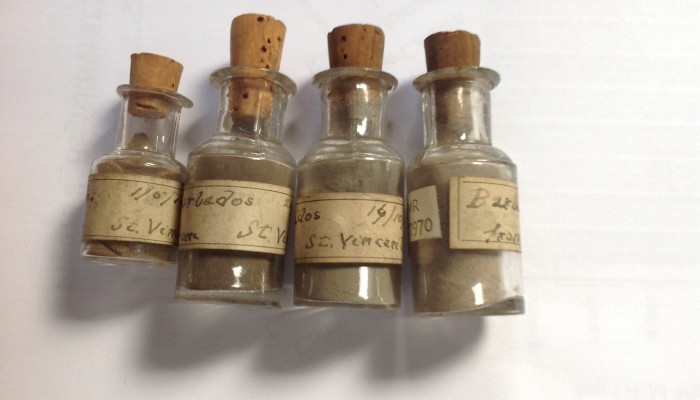
There’s not much that beats the thrill of discovery.. particularly when it turns up in your own backyard. This summer, I have been on the hunt for records and reports of the 1902 eruptions of St Vincent, a lush volcanic island in the Eastern Caribbean. There are indeed many reports from this eruption, carefully documented in official records from the time. But, more surprisingly, there are ...[Read More]
The eruption of Kelut, Java, February 2014
I have used storify.com to put together a synopsis of the February eruption of Kelut, Java, Indonesia. There are some additional links to more detailed posts and related information below. Related posts Fascinating technical analysis of the satellite data from Nicarnica Aviation Erik Klemetti on Wired Volcano Discovery updates on Kelud Satellite imagery collated by Imageo on Discover Magazine Coll ...[Read More]
August Anniversaries: the eruption of Krakatoa
August 27th marks the anniversary of the culmination of the great eruption of Krakatoa (or Krakatau) in Indonesia in 1883. This devastating eruption has become the archetype of a volcanic catastrophe, even though it was a geologically modest example of a ‘caldera forming’ event. The eruption of Krakatoa quickly made the headlines around the world, in part because newly installed unders ...[Read More]
Timelapse volcanoes in Google’s Earth Engine
With the marvels of technology and the generosity of Google and NASA, we can now sit back and watch the back catalogue of volcanic eruptions using the magnificent Google Earth Timelapse of Landsat images. Here are just a few that I have picked out.. Enjoy, and do send more suggestions! Anatahan, Marianas, erupted in 2005. Anatahan Timelapse Chaiten, Chile. Erupted in May 2008: look for the splash ...[Read More]
Santiaguito Volcano: Ninety Years and Counting.
Santiaguito volcano, Guatemala, burst into life in 1922 and is now the second longest continuing eruption. It has outlasted both Stromboli (Italy) and Sangay (Ecuador), both erupting since 1934, and is only outdone by Yasur (Vanuatu), which has been erupting at least since 1774, when first visited by Captain Cook. These long-lived eruptions give us an unusual opportunity to use the slowly-extruded ...[Read More]
Chilean volcanoes: shaken, but not always stirred?
November 7th marked the 175th anniversary of one of the largest earthquakes to have struck northern Patagonia. The earthquake, which is estimated to have had a magnitude of 8, had an epicentre close to Valdivia, and was accompanied by significant ground shaking and subsidence as far south as Chiloe island, and a major tsunami that reached Hawaii. The eyewitness reports of the time have been well ...[Read More]