Archaeopteryx lithogaphica is probably the most iconic dinosaur ever. When it was first discovered, it was heralded as the holy grail of palaeontological findings, as it helped to consolidate the evolutionary continuum between theropod dinosaurs and modern birds. What it also represents though, is an example of the evolution of scientific thought through time. Palaeontologists, mechanists and developmental biologists have long puzzled about where exactly this ancient-winged half-bird half-dinosaur fits into the evolutionary tree of life. Perhaps of equal importance, is what it can tell us about the evolution of one of the most extraordinary biological innovations of all time – the development of wings and powered flight.
A new study by a global team of scientists has added yet another page to the complex evolutionary tale of how Archaeopteryx, and it’s not so distant cousin, Anchiornis huxleyi, used their feathers as an adaptation for aerial motion, or flight – whatever you want to call it. The position was long held that the arrangement of feathers in Mesozoic feathered dinosaurs, including birds, was the same as that in modern birds, the Neornithes; a layer of long asymmetrical flight feathers overlain by short covert feathers (it’s the asymmetry of these feathers that generates lift). New imaging techniques used by the team, however, have uncovered hitherto missed out complexities in the arrangements and structures of these feathers in Anchiornis and Archaeopteryx.
Archaeopteryx and Anchiornis appear to have represented early evolutionary experiments in feather configuration, differing from Neornithines by having multiple layers of feathers. In Archaeopteryx, primary feathers are overlain by dorsal (above) and ventral (below) coverts, and in Anchiornis the configuration is similar, but slightly more primitive by having short, slender, and symmetrical remiges, with adjacent secondary feathers not strongly overlapping. These layers are arranged into four tiers overlying the primary feathers, which decrease in length systematically with each progressive layer.
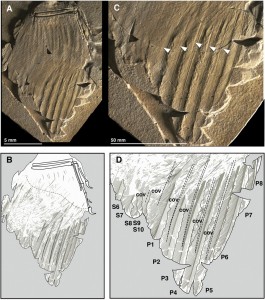
Right wing of Archaeopteryx. A: Ventral view, B: Line drawing, C: Closeup shot, D: Line drawing of primaries and coverts (click for larger: Copyright Longrich et al)
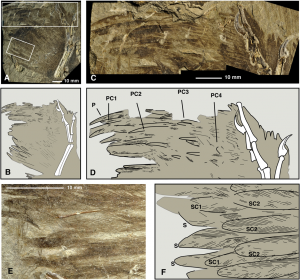
Wing of Anchiornis, A: Left wing, B: Line drawing, C: Detail of outer wing. D: Line drawing, E: Detail of inner wing, F: Line drawing (click for larger image. Copyright Longrich et al)
The two species show remarkably different styles of preservation. Anchiornis, from 160 million year old deposits in Liaoning, China, has feathers preserved through the remains of organic compounds known as melanosomes. These structures an actually be used to identify the colour that these feathers once were when the animal was alive! Archaeopteryx is entirely different. It was first thought that the infamous feather marks were impressions left in the fine sediment on a single plane, but now it is considered that they represent collapsed moulds, passing through the surrounding matrix in three dimensions. This revelation means that the split in the matrix passes through different layers, so you actually get a transitional cross-section through the different layers of feathers, and has led to an entirely new interpretation of the internal geometry and structure of the feathers.
There has been much debate regarding the flight capability of Archaeopteryx and other early avians. Part of this debate stems from uncertainty regarding the mechanical properties that are influential for modelling flight, such as muscle strength, mass, and thickness of internal feather structures. The macro-structure of feathers, and their precise function tied in with all of this, makes it quite a complex question to pick away at, due to the natural uncertainty tied into such modelling approaches. It is likely that the slender feather shafts exhibited by both Anchiornis and Archaeopteryx were unable to support the mass of the animals during sustained flight. However, the realisation that the feathers are arranged in layers suggests that this compensated for this lack of strength, and may have been sturdy and thick enough to function as airfoils, similar in function to modern birds, but based on a completely different design. Overall, it suggests that both organisms would not have been capable of taking off or flying at low speeds, and that instead the primary function of their wings was in high speed gliding or flapping, which lends support to the highly polarised debate between a ‘trees-down’ or ‘ground-up’ origin of flight, in favour of the former.
But why is all of this important? Nature continues to astound us with the most remarkable inventions beyond our wildest imaginations. It took millions of years for the dinosaurian forelimb to become modified, in terms of bone and muscle configuration and feather structure, to become a highly efficient airfoil adapted for flight and with the ability rapidly change its span, shape and area to exploit a wide variety of flight kinematics and aerodynamic functions. It is this transformation that allowed dinosaurs to take to the skies and dominate them for some 150 million years, still capable of taking our breath away today. However, if we were just to look at modern birds, which predominantly have the same feather configuration, we would have no clue as to how this transition occurred, and it’s only thanks to the fossil record that we can get tantalising glimpses into this. What we see is a departure of a primitive Archaeopteryx-ian arrangement, experimentation with Anchiornis and a seemingly unspecialised suite of different arrangements in other feathered dinosaurs, and less than 20 million years later, the total achievement of the modern configuration within Enantiornithes (first identified in Eoalulavis hoyasi), the earliest of which is Protopteryx fengningensis (131 million years old).
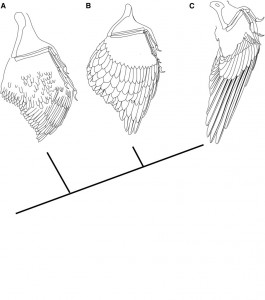
Hypothetical sequence of early evolution of the avian wing, with Anchiornis (left), early avialae such as Archaeopteryx (central), and pygostylians such as Confuciusornis (right) (click for larger, copyright Longrich et al)
Surprisingly, this format of relatively rapid experimentation and development is not only seen within birds, but also in pterosaurs and bats, both of which also show a punctuated-stasis model, with the final attainment of an ideal morphological design retained once found. Even more bizarrely, this pattern is replicated within modern manmade flight engineering! In the early 20th century, there were rapid advances in the designs of aircraft, followed by a period of much slower progress. So are the processes governing this largely the same? Both must obey the constraints of mechanics and fluid (aero-) dynamics and this actually imposes quite tight limitations on the number of geometrically feasible configurations that allow for flight capability. In both evolution and engineering, once these ideal configurations are found, the process of refinement is much slower, and any significant deviations will be functionally compromising. Weird how that works eh.
Guillermo Rein
Using simple fluid dynamic concepts, Octave Levenspiel, renowned engineering professor at Oregon State, has reasoned since 1992 that if we were to accept that big ancient creatures like the Quetzalcoatlus could fly (12-15 m wingspan), then “we have to accept that ancient Earth’s atmospheric pressure was very different from what it is today” (3.2 to 4.8 bar, instead of current pressure of 1 bar).
You can read his short paper “Atmospheric Pressure at the Time of Dinosaurs” here: http://levenspiel.com/octave/OL_images/DinosaurW.pdf (or http://levenspiel.com/octave/dinosaurs.htm)
I know little or nothing about dinosaurs or Earth’s past atmospheric conditions, but I find Prof Levenspiel’s simple use of fundamental laws rather powerful. I wonder what are the arguments
against this other than “We don’t believe in aerodynamic theory”.
tennant
Ooh, thanks, that certainly seems like an interesting thing to look into!
Also, *waves at from the Royal School of Mines*
Mike Taylor
“it’s the asymmetry of these feathers that generates lift.”
Well, no. It’s primarily the shape of the wing, and the flapping motion. Asymmetric feathers help but they’re by no means necessary. (If it were so, then bats and pterosaurs would be unable to fly.)
I’m afraid the Levenspiel paper is a manifestation of this syndrome: http://www.smbc-comics.com/?id=2556
Pingback: Fossil feathers are frickin’ sweet | Green Tea and Velociraptors