Devastating supervolcanoes can erupt simply due to changes that happen in their giant magma chambers as they slowly cool, according to a new study. This finding marks the first time researchers have been able to explain the mechanism behind the eruptions of the largest volcanoes on Earth.
Geologists have identified the roots of a number of ancient and possible future supervolcanoes across the globe. No supervolcano has yet exploded in human history, but the rock record demonstrates how devastating any eruption would be to today’s civilisation. Perhaps most famous is the Yellowstone supervolcano in Wyoming, which has erupted three times in the past two million years (the last eruption occurred 600,000 years ago).
These giant volcanic time bombs seem to explode once every few hundred thousand years, and when they do they throw huge volumes of erupted ash into the sky. At Yellowstone, the eruption that happened two million years ago ejected more than 2000km3 of material – enough to cover Greater London in a mile thick layer of ash.
It is estimated that a super-eruption like that would drive a global temperature drop of 10˚C for more than a decade. Such a dramatic change in global climate is difficult to comprehend. Aside from the instant local devastation, there would be global impacts such as crops failing, followed by large famines.
Despite their potential threat, comparable to a large asteroid impact, the mechanisms and origins of super-eruptions have remained obscure. Modestly sized volcanoes operate on different time-scales and magnitudes, and their eruptions appear to be triggered by pulses of molten rock, magma, which increase the pressure on underground magma chambers that feed their vents.
Two papers recently published in the journal Nature Geoscience try to solve the mystery of how super volcanoes are formed and how they erupt.
Using experiments and computer modelling scientists have discovered what drives a super-eruption. They find that, over time, the underground magma becomes increasingly more buoyant. It is like a beach ball held down beneath the waves until it is released, when it shoots into the air, forced up by the dense water around it.
In the first paper, a team led by Wim Malfait and Carmen Sanchez-Valle of ETH Zurich the Zurich team used a synchrotron, an instrument that can generate intense X-rays, to measure the density, temperature and pressure of molten rock held in a magma chamber several kilometres below the surface. They mimickeed deep Earth conditions in the lab at the European Synchrotron Radiation Facility, which allows probing of substances held at temperatures up to 1,700˚C and the pressure of 36,000 atmospheres.
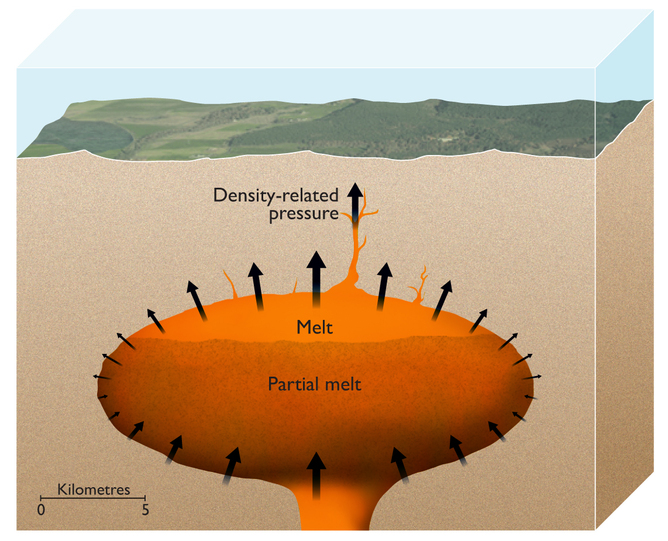
An artist’s impression showing the magma chamber of a supervolcano with partially molten magma at the top. The pressure from its buoyancy is sufficient to punch through 10km or more of the Earth’s crust above it. ESRF/Nigel Hawtin
To feed a supervolcano you need a huge magma chamber. The Zurich team’s results show that as the magma chamber cools it begins to solidify and crystals grow in it that are denser than the magma, which then fall to the base of the chamber. In contrast, the remaining molten rock in the chamber gets progressively less dense, and, if there is enough of it, their measurements showed that the magma eventually becomes light enough that it forces its way through more than 10km of Earth’s overlying crust.
Co-author Carmen Sanchez-Valle, also at ETH Zurich, said: “Our research has shown that the pressure is actually large enough for the Earth’s crust to break. As it rises to the surface, the magma will expand violently, which is a well known origin of a volcanic explosion”.
The second paper by Luca Caricchi and colleagues at the University of Bristol, describes computer simulations of the same processes, finding that the buoyancy of melt in maturing magma chambers is also key to these huge events.
Supervolcanoes require a steady accumulation of molten rock that remains hot enough that it does not completely solidify. It is then simply a matter of time. Malfait’s data show that eventually buoyancy alone is sufficient to trigger these rare, but massive, geological catastrophes.
The eruption of massive supervolcanoes seems to be an inevitable part of their “life cycle”. Just as a star may eventually become a supernova, so a huge magma chamber can eventually become a massive eruption. This contrasts with the way that more familiar smaller volcanoes erupt, where blasts follow directly from rapid injections of magma, or from earthquakes that might trigger them, or even from pressure release on melting of overlying glaciers, as seen in Iceland recently.
This article was originally published at The Conversation.
Read the original article.