Marion Ferrat takes a look under the frozen layers of Arctic permafrost and discusses how these soils may come back to haunt us.
The vast plains of Siberian or Canadian permafrost are a sight to behold. Hundreds, sometimes thousands of miles of frozen soils cover these lands, a cold and barren environment. In places, however, this permafrost is slowly melting away as a result of rising temperatures. The problem with that is that permafrost contains carbon, a whole lot of carbon, about the same amount as is present in the atmosphere today. At the moment, this carbon is fixed inside on- and offshore frozen soils but researchers fear that permafrost melting could suddenly release it into our atmosphere. With CO2 emissions still on the rise and global temperatures steadily increasing, eyes have slowly turned towards these frozen carbon pools.
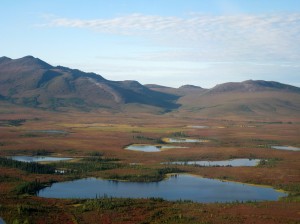
Permafrost and Arctic lakes of the Kobuk River valley, Alaska. Source – 16Terezka, Wikimedia Commons.
A comment published last month in the journal Nature woke many people up to the question of permafrost. The authors, experts in climate modelling, policy and management, estimated the impact on the global economy of a sudden methane release (or methane ‘pulse’) from thawing of offshore permafrost. Their results were rather explosive: the authors put an astounding $60 trillion price tag on thawing of the East Siberian Arctic Shelf permafrost over the next decades, labelling it an ‘economic time bomb’. Most of this cost will be borne by developing countries, they added. Now this is rather worrying.
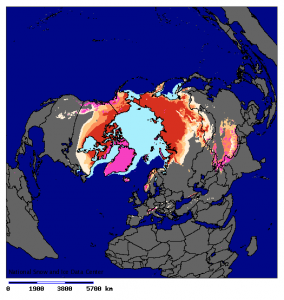
Location of Northern Hemisphere permafrost. Glaciers and ice sheets are in violet and sea ice in light blue. Source – NSIDC, Wikimedia Commons.
The article caused quite a bit of commotion in the news world, with a flurry of articles showing varying degrees of agreement. It was discussed in The Guardian, including an interview of study author Prof Peter Wadhams, The New York Times and The Carbon Brief, amongst other sources. The Washington Post published a fiery criticism of the research, calling it a “misleading commentary”, which spurred a reply from Prof Wadhams.
The problem with simulating the impacts of permafrost thawing is that it is a very complex problem involving many components of the Earth system. I have experienced this first hand as a postdoctoral researcher in Beijing, desperately trying to improve permafrost simulations in my university’s model.
First of all, what exactly is permafrost?
The exact definition of permafrost is a layer of soil that remains at or below 0°C for at least two consecutive years. The uppermost layer of permafrost soils, what we call the active layer, is most sensitive to surface temperature changes and actually goes through an annual cycle of freezing in winter and thawing in summer. Below this active layer is the true permanently frozen permafrost, with all its estimated 1466 Gton (that’s one billion tons) of stored carbon. Yup, that’s quite a lot.
Where does all the carbon come from?
Our present day ice sheets were born during the last glaciation, between approximately 110,000 and 12,000 years ago. The peak of this glacial age around 22,000 years ago, when ice extent was largest, is called the Last Glacial Maximum. Permafrost also dates from this colder and dryer time. The frozen soils of the glaciation, with all their frozen leaves, plants and other sources of organic carbon, were slowly buried by sedimentation processes such as dust deposition from the atmosphere, alluvial deposition (the deposition of eroded loose sediment on land) and peat growth. This increased the thickness of the frozen soil, effectively locking away the carbon.
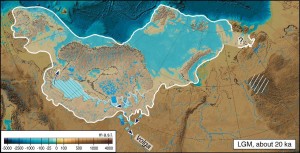
Ice extent in Eurasia during the Last Glacial Maximum. Source – Mangerud et al. (2004), Wikimedia Commons.
What happens when permafrost thaws?
Worms and other burrowing creatures are not the only inhabitants of soils. Deeper underground also live plenty of microscopic microbes, happily munching away at our precious carbon. Microbes, like us, release carbon when they breathe, a process called respiration. When soil becomes permafrost, microbial activity stops. This is what effectively removes the organic carbon from the carbon cycle, making it “unusable”. As permafrost thaws, microbial activity resumes, mixing the carbon and slowly moving it up towards the surface, where other living organisms will then contribute to releasing it to the atmosphere by respiration. This is only the first worry. Another big question is whether this carbon will be released as carbon dioxide (CO2) or methane (CH4). Methane is a less common but much more potent greenhouse gas, so the effect of a sudden and large-scale methane ‘pulse’ would be much more dramatic than a similar CO2 emission.
What are the difficulties in modelling permafrost?
Modelling permafrost evolution and associated carbon releases is difficult because climate models must be able to accurately simulate many different processes: land-atmosphere-ocean interactions, air temperatures, soil temperatures and intricate biogeochemical processes linked to respiration, all at once. That is no easy feat.
Now for all of these different aspects, we also need some measured data. The key to using climate models in general is that, before they can be used to make predictions, they need to be verified against real world data that has been directly measured on Earth. That is, modellers first try to get their models to reproduce what they already know. Only when they are satisfied that they can simulate the past and the present do they start to model the future.
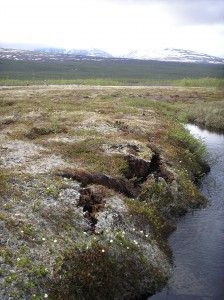
Permafrost peatbog border in Storflaket, Abisko, Sweden. Source – Dentren, Wikimedia Commons.
Given the complexity of modelling permafrost, this means that we need a whole lot of data to verify our models. Air temperature is well recorded around the globe but we also need both spatial and temporal information on soil temperatures, microbial respiration and carbon fluxes from the ground to the atmosphere. Obtaining a good global coverage of all of these factors takes time, especially when we are talking about an annual process like permafrost thawing. More and more papers have been published in recent years and have populated our global dataset of permafrost-related data. Just last month, a study published in Nature provided new data on changes in the carbon stock in Greenland permafrost from 1996 to 2008, as well as results from laboratory thawing experiments.
Dr Kevin Schaefer, from the National Snow and Ice Data Centre (NSIDC), is a bit of a pioneer in permafrost modelling. He helped me when I was working on improving my soil temperature model in Beijing and showed me how intricate the problem was and how much still needed to be improved to accurately simulate permafrost dynamics. He and his colleagues have been working on all aspects of permafrost science for some years now and their papers can provide much information on recent developments.
So the complex nature of permafrost, and the relative novelty of including permafrost in models, is why the range of estimates is still relatively wide. As with every type of model result, this is not to say that scientists disagree on the fundamental processes: there is plenty of frozen carbon, the Earth is warming, if it continues to do so the permafrost will thaw, thawing will eventually lead to a carbon release. We know it happened in the past (see this study published in Nature last year) and it can happen again.
The only question in my opinion is will it be in the next few decades or the ones after that. Many groups around the world are working on measuring, monitoring and modelling permafrost and there is yet to be published a comprehensive, scientific review paper on the latest results of these works. Perhaps such a review would be helpful to communicate the state of our knowledge to the public and policy-makers, and draw attention to yet another part of the Earth system, which will surely be affected by our increasing emissions and transform our world in return.
Marion Ferrat
Kate G
I really enjoyed the article! Can you elaborate a little on the chemistry behind why carbon might be released as CO2 versus methane, and why this is difficult to determine?
Marion Ferrat
Thank you very much for your comment and kind feedback Kate! Glad you enjoyed the article. 🙂
When microbial activity resumes, the thawed carbon can be converted by different types of bacteria either into CO2 or CH4, bi-products of their metabolism. From my understanding of the biology, the microbes that produce methane (methanogens) also need other microbes to first convert the plant material and carbon into simple molecules. It’s a bit like a giant micro food chain (I know that’s a bit of an oxymoron)! These processes are likely influenced by factors such as temperature, pH and water content amongst other things and their effects are difficult to determine because a lot of parameters need to be known..
Some more information about biological processes of CO2 and methane production by microbes can be found below:
http://micro.cornell.edu/cals/micro/research/nsf-observatories/yavitt-zinder/organic-matter.cfm
http://www.iea-coal.org.uk/documents/82405/7606/Microbial-methane-from-carbon-dioxide-in-coal-beds-(CCC/174)
Hope this helps!