As Earth’s environment changes, it leaves behind clues used by scientists to paint portraits of the past: scorched timber, water-weathered shores, hardened lava flows. Chile’s Conguillío National Park is teeming with these kind of geologic artifacts; some are only a few years old while others have existed for more than 30 million years. The photographer Anita Di Chiara, a researcher at Lancaster U ...[Read More]
Imaggeo on Mondays: Chilean relics of Earth’s past
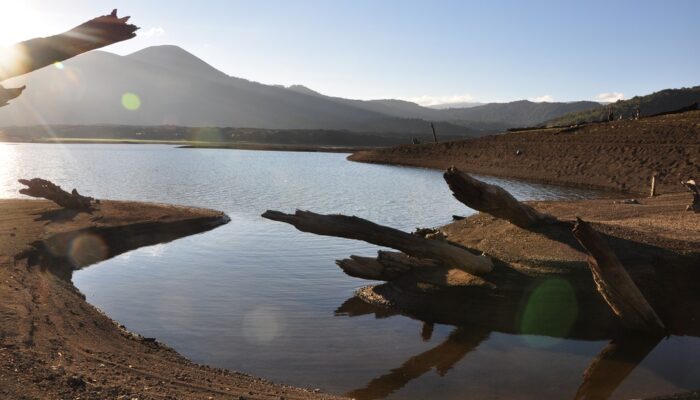