In 1821, peat cutters discovered a body similar to a mummy, pinned down by two wooden stakes deep in the mud. The body’s face still held red hair and a beard, their teeth were well preserved, and a hoop of willow was wrapped around their throat. But this wasn’t the dry, hot climate of Egypt but a cold and rain-sodden bog of Ireland. Later assessment suggested that these were the remain ...[Read More]
Imaggeo On Monday: Marble quarry west of Alicante, Spain
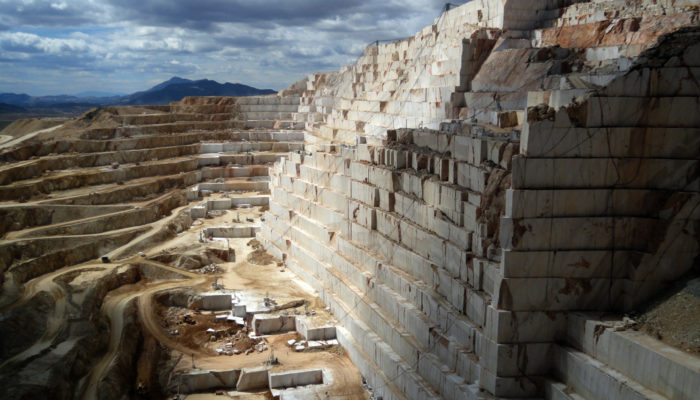
The largest marble quarry in Europe is located at Monte Coto next to Pinoso in the Spanish Alicante province. Here an Eocene limestone named “Crema Marfil” is exploited on a large scale. Photo by Christoph Mayr, as described on imaggeo.egu.eu. Imaggeo is the EGU’s online open access geosciences image repository. All geoscientists (and others) can submit their photographs ...[Read More]
Imaggeo On Monday: Works of art – works of muck
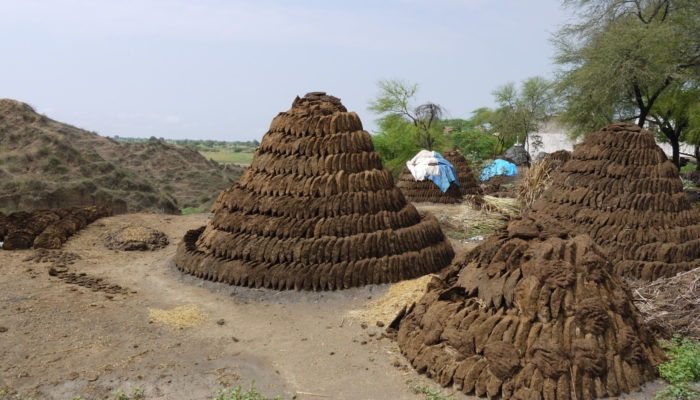
Dung cakes made from buffalo manure are artfully piled up in a village in Madhya Pradesh. Used as fuel for cooking fires, they are a sustainable energy source in rural areas of India, but add to air pollution by biomass burning. Photo by Irene Marzolff, as described on imaggeo.egu.eu. Imaggeo is the EGU’s online open access geosciences image repository. All geoscientists (and others) ...[Read More]
Imaggeo On Monday: Beech leaves on burned ground
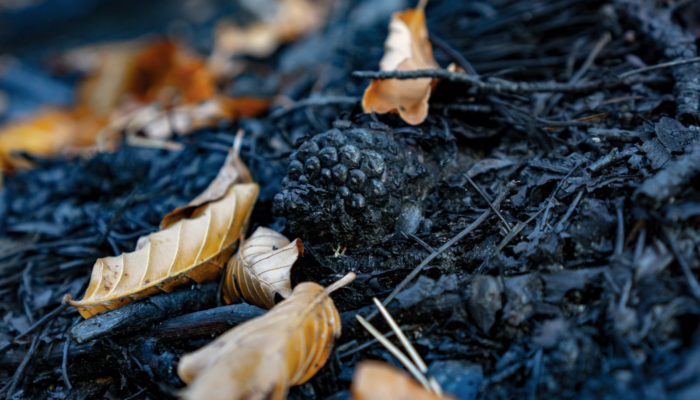
A charred pine cone lies on the ground of a mixed beech-pine forest following a forest fire in the Rax-Schneeberg region of Austria in 2021. The charred pine cone and litter are surrounded by unburned yellow beech leaves that fell to the ground after the surface fire. Pyrogenic carbon such as the charred pine cone can remain stable in the environment for extended periods of time and can affect bio ...[Read More]