Profile of a specimen of Arctica islandica, one of the longest lived marine bivalves known, undergoing a 3D scan. Their longevity is exploited for reconstruction of climate patterns in the North Atlantic. However, mathematical models of their morphodynamics are necessary to account for bias induced by their asymmetric growth (“morphodynamics” is the study of how an organisms’ gro ...[Read More]
Imaggeo On Monday: Studying shell morphodynamics to improve climate models
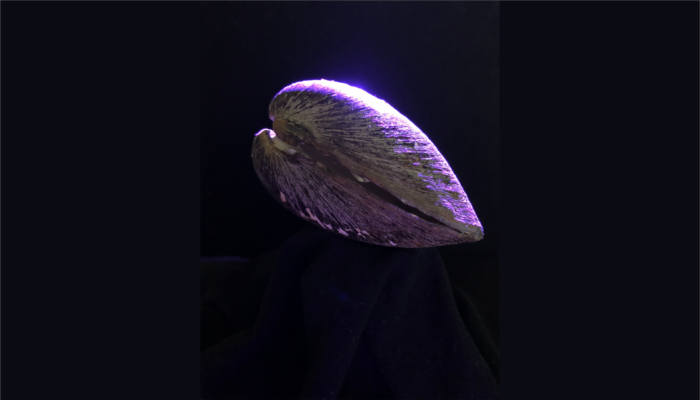