These delicate ice structures may look like frozen honeycombs from another world, but the crystalline patterns can be found 80 degrees south, in Antarctica, where they are shaped by the white continent’s windy conditions. In Western Antarctica is a 9-kilometre line of rocky ridges, called Patriot Hills. Often cold wind furiously descends from the hills across Horseshoe Valley glacier, sculpting do ...[Read More]
Imaggeo on Mondays: Antarctic winds make honeycomb ice
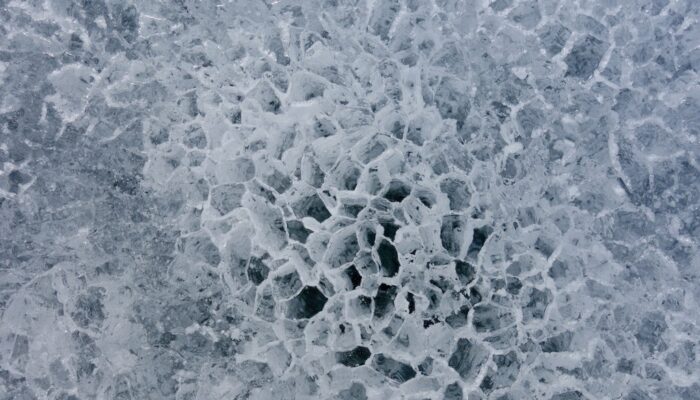