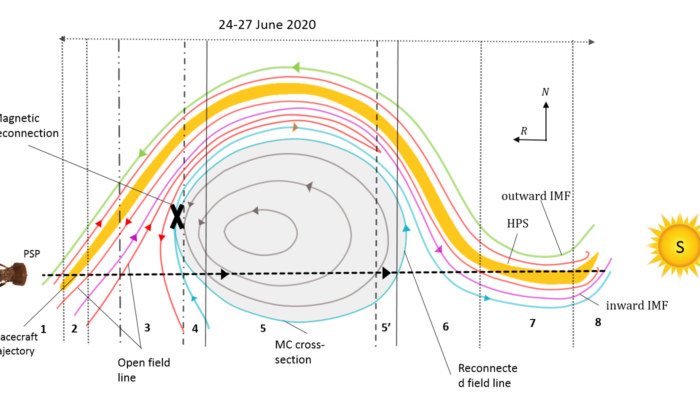
In the early 1970s, when Coronal mass ejections (CMEs), massive eruptions of magnetized solar plasma into interplanetary space, were first discovered, they were assumed to be always associated with the energetic solar phenomenon like solar flares, prominences, etc. With improved coronagraphic and multi-wavelength observational instruments, a class of CMEs emerging from the streamers was found and named Streamer-blowout CMEs (SBO-CMEs; Vourlidas et al. 2018). These CMEs have signatures of flux ropes but have no direct association with solar active regions, flares, and filaments. Thus, they lack classic low-coronal signatures and are characterized as stealth CMEs (Robbrecht et al. 2009). Nonetheless, with advanced image processing, geometric techniques, and at least one secondary viewpoint, recent studies can identify weak eruptive signatures for stealth CMEs (Palmerio et al. 2021a). Stealth CMEs have significant importance in space weather since they can cause significant geomagnetic storms whose magnetic properties are hard to predict. Occasionally, they are the solar counterparts of the so-called ‘problematic geomagnetic storms.’
A solar eruption such as a CME follows a gradual accumulation of free magnetic energy and subsequent triggering of plasma instabilities in energized solar magnetic structures. The accumulated free energy can power consecutive sympathetic eruptions from a multipolar flux system (Li et al. 2008). In a sympathetic eruption, the eruptive flare reconnection of the first CME simultaneously acts as an overlying pre-eruption breakout reconnection (Antiochos et al. 1999) for the second one (Lynch and Edmondson 2013). Using an MHD simulation, (Torok et al. 2011) showed that sympathetic eruption from a flux system of a coronal pseudostreamer could be triggered by a prior eruption that occurred outside but adjacent to the pseudostreamer.
Revealing erupting solar sources
In one of our recent studies, with the help of extrapolated coronal fields and multiple viewpoint remote observations, we investigated the initiation and formation of a classic SBO-CME (CME #2) that was preceded by two consecutive CMEs (CME #0 and CME #1). Moreover, the initiation mechanism was consistent with the multi-stage, sequential (and most likely sympathetic) eruption scenario (Lynch and Edmondson 2013). Upon analyzing the extreme ultraviolet (EUV) on disk and off limb solar observations obtained from the two viewpoints being 70-degree apart from each other and the potential field source surface (PFSS; Wang and Sheely 1992) representation of the global magnetic field, we located the regions associated with the initiation of the CMEs on the solar disk. In Figure 1a, an EUV difference image of a solar disk is shown where the areas related to CME #0, #1, and #2 are indicated with squares. Out of the three CMEs, CME #0 had an easily recognizable on-disk low coronal signature – coronal brightening (marked with a pink box on Fig 1a) that was located immediately outside (northwest) of an equatorial multipolar flux system (marked with a red box) associated with the eruption of CME #1 and #2.
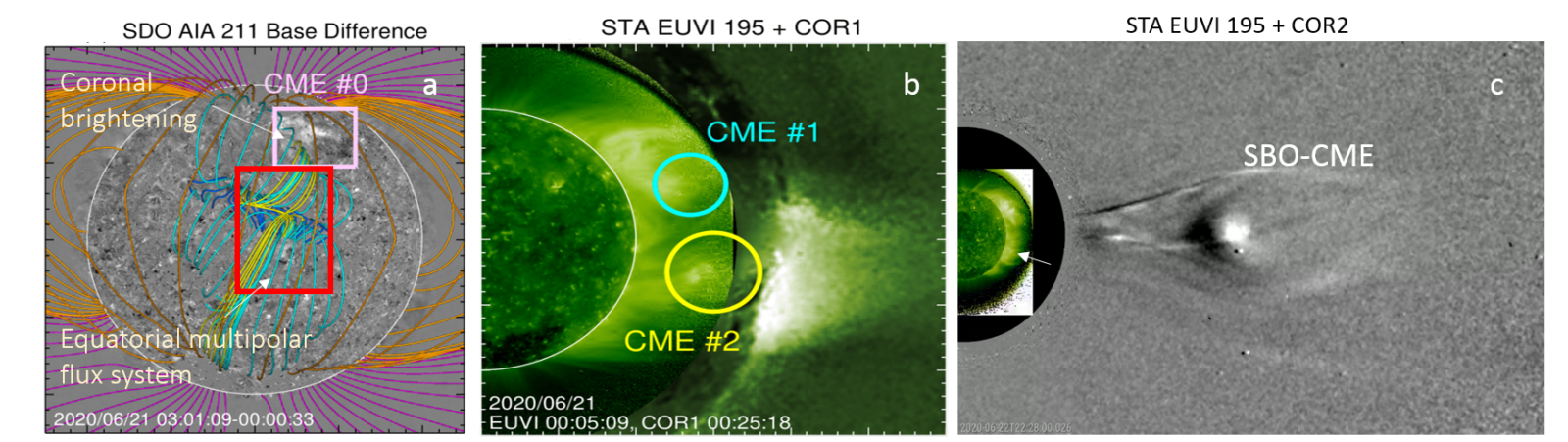
Figure 1: Solar counterparts of the SBO-CME and its preceding sequential eruptions. a) The coronal signature of CME #0 and the multipolar flux system originating CME #1 and #2. b) Coronal regions related to CME #1 and #2. c) The classic SBO-CME observed by off-limb coronagraph. The arrow denotes the coronal region related to the SBO-CME.
Eruption initiations
Our observational analysis suggests that a direct and causal magnetic coupling between these eruptions exists. The first sequential eruption, CME #0, triggered the sympathetic eruption from the adjacent energized multipolar flux system. It removed a portion of the overlying helmet streamer flux that eventually led to disruptions of the quasi-equilibrium force-balance condition of the nearby multipolar flux system. Ultimately, this resulted in the sympathetic eruption of CMEs #1 and #2 from the multipolar flux system’s northern and southern side lobe arcades, respectively (see the marked regions in Figure 1b). Both sympathetic CMEs deflected towards their overlying breakout current sheet corresponding to the local pressure minima (Lynch and Edmondson 2013). CME #2 appeared as an SBO-CME and gradually deflected northward towards the heliospheric current sheet (HCS) — a boundary between open and oppositely directed heliospheric field lines. Figure 1c shows the classic SBO-CME structure observed using off-limb STEREO (Solar TErrestrial RElations Observatory) A coronagraph. Figure 1a and 1b are reproduced from Fig 2-3 in Pal et al. 2022 accepted by Frontiers in Astronomy and Space Sciences.
The heliospheric evolution
Being of extremely slow speed (~220 km/s), the stealthy SBO-CME took ~8 days to reach 1 AU. It was observed in situ with a triple lineup by the Parker Solar Probe (PSP, heliocentric distance R~0.5), BepiColombo (R~ 0.88 AU), and Wind (1 AU) spacecraft, where except PSP, the other two spacecraft crossed the interplanetary part of the CME (ICME) almost through its flank (Möstl et al. 2022). In the in-situ observations, the CME appeared as a low inclination, right-handed, and South-West-North magnetic flux rope (FR). The continuous probing of the solar wind preceding and following the ICME FR reveals that large-scale magnetic structures draped about the ICME while reaching 0.5 AU. Draping of interplanetary magnetic fields (IMFs) about ICMEs is a typical process in the heliosphere (Mccomas et al. 1988), where the pattern of the draping depends on the ICME sizes, shapes, and speeds relative to the upstream solar wind plasma (Gosling and McComas 1987). The draped IMFs may interact with the ICMEs and lead to a tail-like structure with almost constant magnetic field components at the back of ICME FRs (Dasso et al. 2007, Pal et al. 2020, 2021a, Pal 2021b).
Upon analyzing the in-situ observations of the solar wind plasma surrounding the ICME FR (hereafter magnetic cloud (MC)) by PSP, we revealed the interplay between the slow ICME and its surroundings in the inner heliosphere (R~0.5 AU). Careful observations of solar wind speed, magnetic field intensity and coherent rotation of its vector components, plasma density, temperature, gas pressure to the magnetic pressure ratio, and the suprathermal electron pitch angle distribution obtained from PSP, we inferred that the spacecraft came across the outward and inward-directed IMFs along with the heliospheric plasma sheet (HPS) encasing the HCS, before and after its encounter with the MC. PSP found that the HCS changed its inclination to the PSP orbital plane from its high (~ 40 degrees) value to a low (~ 29 degrees) value as the spacecraft crossed through the ICME surrounding structures. The PSP observational analysis showed that the heliospheric magnetic field and plasma sheet structures draped about the ICME, and the draped heliospheric field lines reconnected with the MC at its front. The magnetic reconnection at the MC front resulted in a tail-like magnetic structure behind the MC populated with reconnected field lines. At R~0.5 AU, PSP witnessed a loss of ~ 20% of the MC azimuthal magnetic flux that the MC lost due to magnetic reconnection that initiated after a heliocentric distance of ~ 0.35 AU. In Figure 2, we provide a schematic of the ICME cross-section and the pattern of large-scale magnetic structure draping about the ICME obtained based on the PSP observation. The different large-scale structures that PSP encountered are indicated by numbers (1-8). The image is reproduced from Fig 6 in Pal et al. 2022 accepted by Frontiers in Astronomy and Space Sciences.
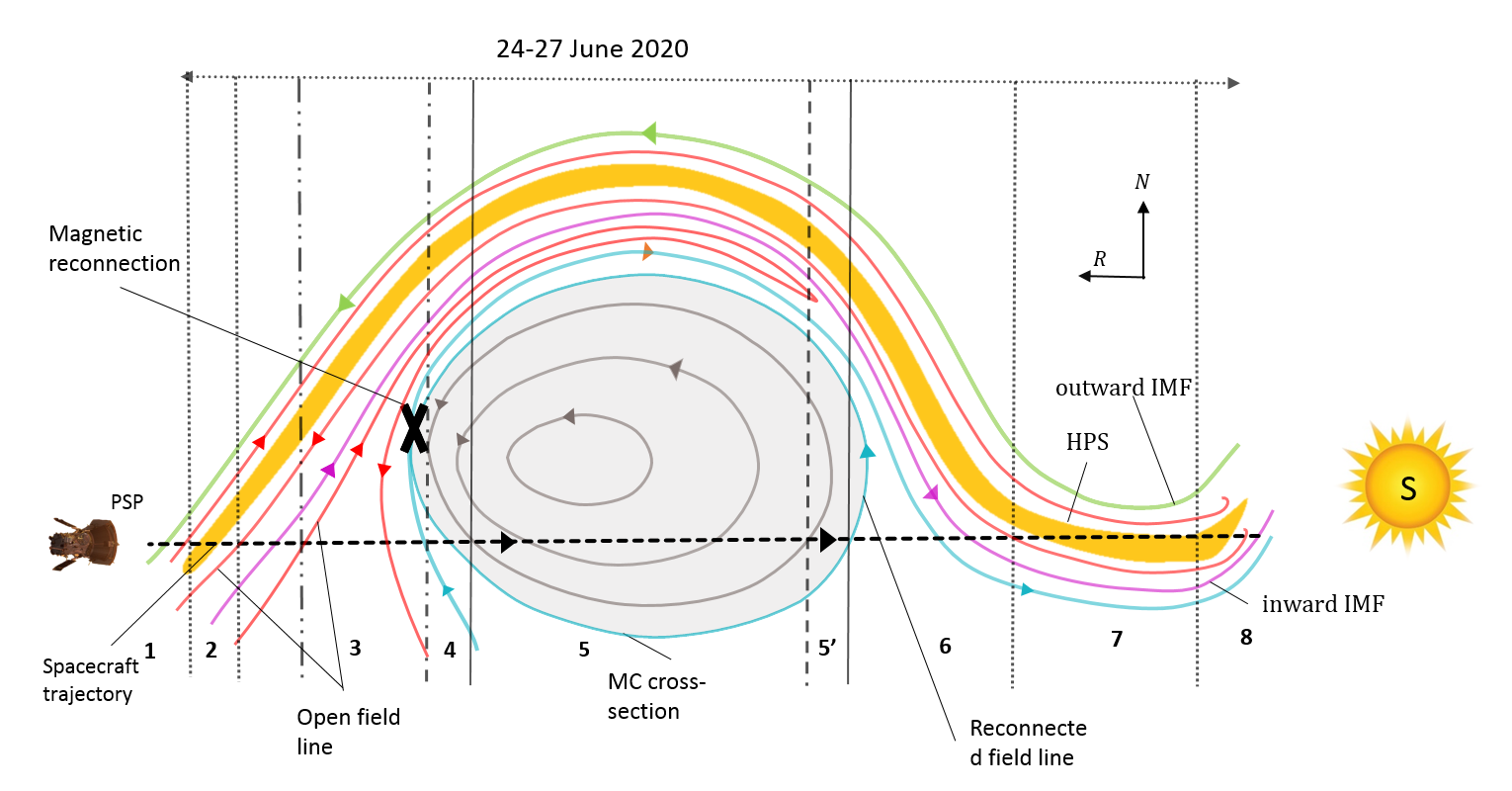
A schematic of the ICME flux rope cross-section and the large-scale magnetic structure draping about the ICME obtained based on the PSP observation.
Several other important aspects in studying this eruption
Remote and in situ multi-viewpoint near-Sun and heliospheric observations and analysis of such a classic stealth streamer-blowout CME has importance from several distinct aspects. Palmerio et al. 2021b evaluated the CME’s early evolution and forward modeled its magnetic configuration. By comparing with the PSP observation in the inner heliosphere, the study showed how the magnetic configuration of a stealthy CME can be reliably predicted. Möstl et al. 2022 analyzed the same event with the triple lineup observations by PSP, Bepi, and Wind spacecraft and suggested the importance of such lineup observations in studying CME’s deformation and progressive erosion. In addition, our analysis of this event contributed to a better understanding of a classic SBO-CME initiation and its interplanetary interactions with the surrounding heliospheric environment. The study has been accepted by the Frontiers in Astronomy and Space Sciences as a part of the research topic ‘Flux Rope Interaction with the Ambient Corona: from Jets to CMEs’ and openly available at http://arxiv.org/abs/2205.07713.
References:
Vourlidas, Angelos, and David F. Webb. “Streamer-blowout coronal mass ejections: their properties and relation to the coronal magnetic field structure.” The Astrophysical Journal 861.2 (2018): 103.
Robbrecht, Eva, Spiros Patsourakos, and Angelos Vourlidas. “No trace left behind: STEREO observation of a coronal mass ejection without low coronal signatures.” The Astrophysical Journal 701.1 (2009): 283.
Palmerio, Erika, et al. “Investigating remote-sensing techniques to reveal stealth coronal mass ejections.” Frontiers in Astronomy and Space Sciences (2021a): 109.
Palmerio, Erika, et al. “Predicting the Magnetic Fields of a Stealth CME Detected by Parker Solar Probe at 0.5 AU.” The Astrophysical Journal 920.2 (2021b): 65.
Li, Y., et al. “The solar magnetic field and coronal dynamics of the eruption on 2007 May 19.” The Astrophysical Journal 681.1 (2008): L37.
Antiochos, S. K., C. R. DeVore, and J. A. Klimchuk. “A model for solar coronal mass ejections.” The Astrophysical Journal 510.1 (1999): 485.
Lynch, Benjamin J., and Justin K. Edmondson. “Sympathetic magnetic breakout coronal mass ejections from pseudostreamers.” The Astrophysical Journal 764.1 (2013): 87.
Török, T., et al. “A model for magnetically coupled sympathetic eruptions.” The Astrophysical Journal Letters 739.2 (2011): L63.
Wang, Y-M., and N. R. Sheeley Jr. “On potential field models of the solar corona.” The Astrophysical Journal 392 (1992): 310-319.
McComas, D. J., et al. “Interplanetary magnetic field draping about fast coronal mass ejecta in the outer heliosphere.” Journal of Geophysical Research: Space Physics 93.A4 (1988): 2519-2526.
Möstl, Christian, et al. “Multipoint Interplanetary Coronal Mass Ejections Observed with Solar Orbiter, BepiColombo, Parker Solar Probe, Wind, and STEREO-A.” The Astrophysical Journal Letters 924.1 (2022): L6.
Gosling, J. T., and D. J. McComas. “Field line draping about fast coronal mass ejecta: A source of strong out‐of‐the‐ecliptic interplanetary magnetic fields.” Geophysical Research Letters 14.4 (1987): 355-358.
Dasso, Sergio, et al. “Progressive transformation of a flux rope to an ICME.” Solar Physics 244.1 (2007): 115-137.
Pal, Sanchita. “Uncovering the process that transports magnetic helicity to coronal mass ejection flux ropes.” Advances in Space Research (2021b).
Pal, Sanchita, Soumyaranjan Dash, and Dibyendu Nandy. “Flux erosion of magnetic clouds by reconnection with the Sun’s open flux.” Geophysical Research Letters 47.8 (2020): e2019GL086372.
Pal, Sanchita, et al. “Uncovering erosion effects on magnetic flux rope twist.” Astronomy & Astrophysics 650 (2021a): A176.