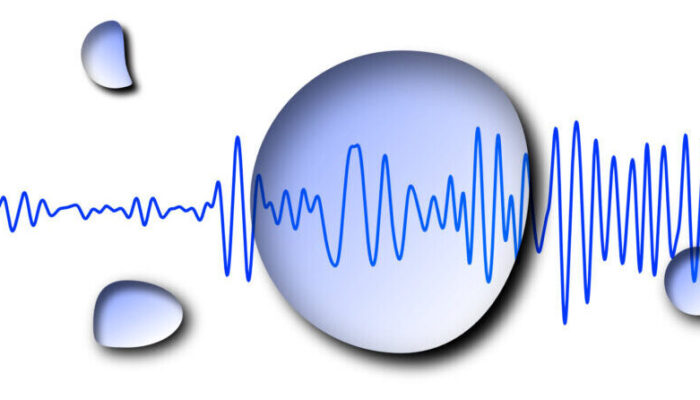
With this article, authors Richard Kramer, Charlotte Bruland, Laura Ermert, Yang Lu and Steffen Birk would like to draw your attention to an exciting topic and their EGU 24 session Hydro-Seismology: seismic insights into Water Resources in the context of climate change!
Critical Resource – The water cycle under the influence of the climate change
The balance of our planet’s ecosystems is tied to the water cycle, an interplay of processes profoundly impacted by climate change. This cycle combines the movement of water on, above, and below the surface of the Earth. It involves processes like evaporation, condensation, precipitation, and runoff, constantly redistributing water. This cycle is not only important for humans offering a renewable source of drinking water vital for health, supporting agriculture and food and energy production, it also plays a crucial role in the ecosystems of our planet.
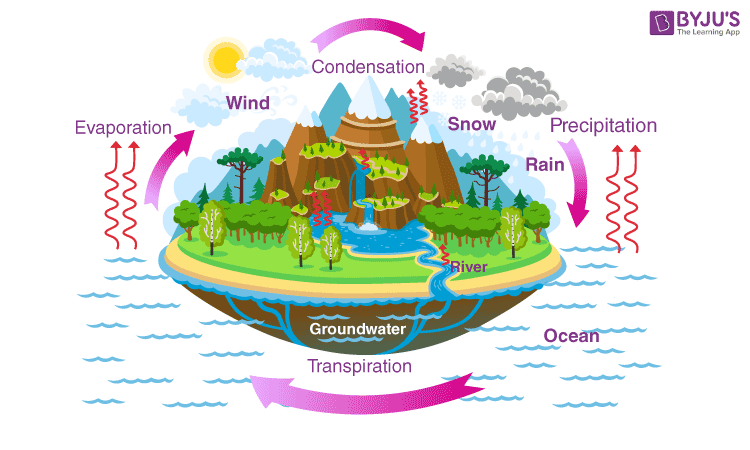
Figure 1: The water cycle process, image CC from https://byjus.com/chemistry/water-cycle-process/
In recent years, the scientific community has increasingly recognized the influence of climate change on the driving mechanisms of our planet’s hydrologic systems. This will lead to alterations in precipitation patterns, the intensity of storms, and the frequency of extreme weather events and it will directly impact groundwater recharge and availability. This bears immense significance for groundwater management, a critical aspect of water resource sustainability in the face of global environmental transformations.
Hydro-Seismology: a complementary view on the hydrological system
Using seismological methods to approach hydrological information has a long history. Firstly, active seismic methods using a powerful source, such as an explosives or vibroseis truck, were used to locate potential groundwater aquifers. These methods were and are still quite expensive and can disturb the environment. With the beginning of the new century a new branch of seismology was invented. This new concept called ambient noise seismology, is independent of sparse and randomly occurring earthquakes and uses sources which are provided by the Earth and its inhabitants. The ocean is a powerful source of ubiquitously and continuously observable low-amplitude seismic waves (Ardhuin et al., 2015). In an effort to also tap higher-frequency sources with known locations, researchers started to utilize seismic signals from opportune anthropogenic sources such as trains for Earth observations too (Brenguier et al., 2019). Ambient seismic noise enables seismologists to monitor the Earth’s subsurface continuously over a long time and retrieve vital information about its changes and its interferences with the environment. There are two common tools to access information about the subsurface in this way: Time-lapse ambient noise tomography and seismic velocity monitoring.
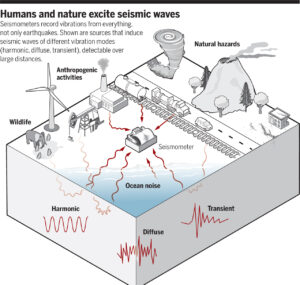
Figure 2: “Humans and nature excite seismic waves. Seismometers record vibrations from everything, not only earthquakes. Shown are sources that induce seismic waves of different vibration modes (harmonic, diffuse, transient), detectable over large distances.
GRAPHIC: N. DESAI/SCIENCE.” From Marine A. Denolle & Tarje Nissen-Meyer (2020).
Already in 2006 scientists were able to link changes in the seismic velocities to precipitation rates and changes in the groundwater table (Sens-Schönfelder & Wegler, 2006). It took some time until the community came back to this approach. Today we are able to use seismological records and processed data for a variety of applications. Seismograms for example provide some first order information about the location and the tracks of large storms in the ocean as well as on land (Retailleau & Gualtieri 2021). On the other hand, we can use these records to recover the seismic wavefields and measure changes which are closely linked to environmental effects and the near surface water content (Voisin et al.2017, Gaubert-Bastide et al. 2017, and Mao et al. 2022).
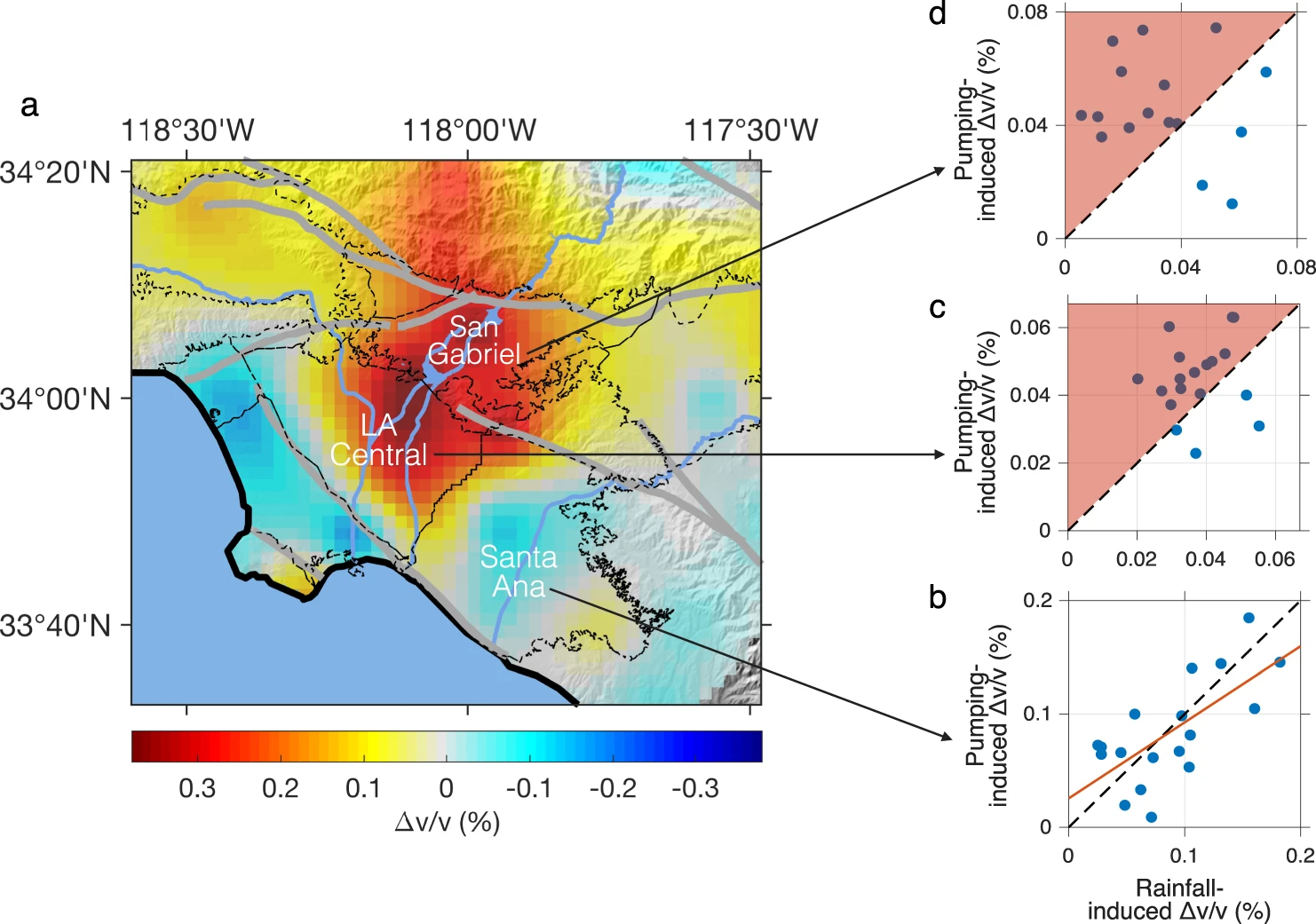
Figure 3: “a Map showing the Δv/v accumulated from 2000 to 2020 (measured in 0.2–0.8 Hz). Colder colors associate with cumulative increases in groundwater and warmer colors with cumulative declines. Note that the boundary between the Santa Ana Basin and the LA Central Basin is a municipal border (i.e., the county line between LA and Orange counties) rather than a natural hydraulic barrier. See Supplementary Fig. 4 for the accumulated Δv/v in 0.5–2.0 Hz (corresponding to shallower depth.) b–d Relationships between two seasonal components of Δv/v (that is, the rainfall-induced decrease in winters and the pumping-induced increase in summers) in b Santa Ana Basin, c LA Central Basin, and d San Gabriel Basin. The rainfall-induced Δv/v are averaged over three-year (backwards) moving windows to downweigh the effect of a few extreme climate events (following the protocol of OCWD). The red line in b denotes the best-fit line (with a slope close to 1).” Mao et al. (2022).
For example, Mao et al. (2022) measured seismic velocity changes in the Los Angeles region. They obtained a nearly perfect match between seismic velocity changes in the Santa Ana basin and the hydraulic head at a well in the basin as well as good fits between InSAR ground deformation maps and seismic velocity changes. Crucially, contrary to InSAR, seismic waves have the potential to provide depth resolution and contrary to point in-situ well measurements, they sense a large area, providing complementary information. Mao and co-workers could also observe long-term trends where recharge and pumping were imbalanced, highlighting different water management practices across municipalities in the Los Angeles Region.
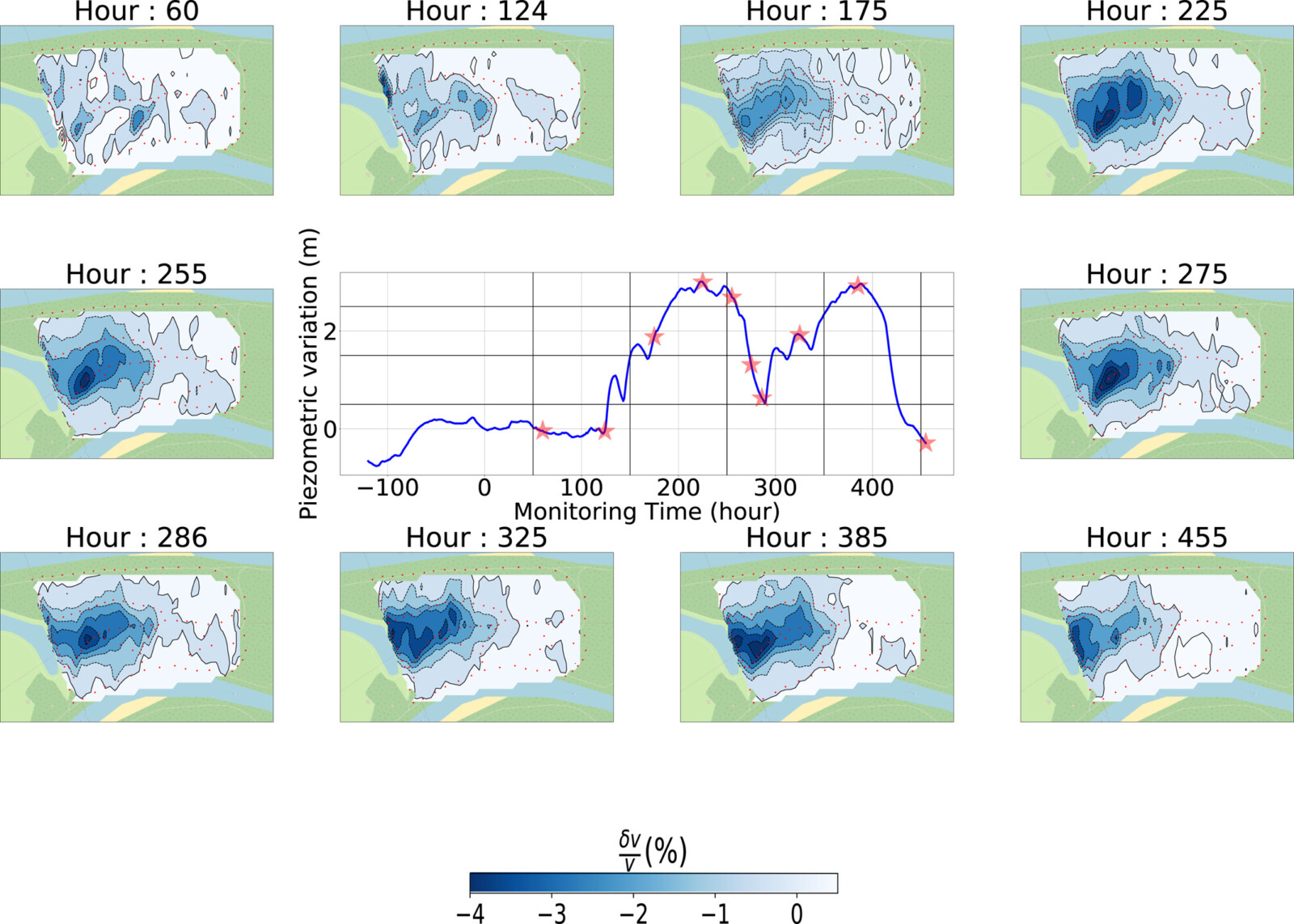
Figure 4: “Evolution of the δv/v maps for 10 different key moments of the controlled monitoring experiments. The central graph shows the relative evolution of the water table measured at piezometer P95. The red stars highlight the moments for which the maps are shown.”, Gaubert Bastide et al. (2022).
Another important aspect in groundwater management is the quality of the water and its protection against pollution. In the future multiple metropolitan areas will face water shortages and have to work on effective strategies to secure the freshwater supply for their inhabitants. A good example to start working on strategies early on is the city of Lyon.
Two studies at Crépieux-Charmy in Lyon (Voisin et al., 2017 and Gaubert-Bastide et al., 2022) examine the effectiveness of a protective hydraulic dome created to shield the city’s drinking water from contamination. Gaubert-Bastide et al. (2022) use a dense seismic network to monitor hourly seismic velocity variations during filling and drainage cycles of an infiltration basin. Their findings image the formation and evolution of the hydraulic dome, revealing insights into water flow between the water table and nearby river. This approach provides a valuable understanding of how well the barrier protects the water field from potential pollution.
The given examples show the capabilities of seismological methods in the context of groundwater monitoring, groundwater management and protection from contamination. While we can only highlight a few selected studies, there are many more out there using different receivers, e.g. fiber optic cables, and targeting different scales, e.g. from lab studies to large scale experiments. They address a variety of questions about water with seismological methods, e.g. the imaging of Karst conduits (ref), the decadal development of ocean wave climate (Aster et al., 2023), groundwater levels (e.g. Clements & Denolle, 2018; Tribaldos & Ajo-Franklin, 2021) and many more.
EGU 2024 – Hydro-Seismology: seismic insights into Water Resources in the context of climate change
If you want to know more about the state of the art research in the field of hydro-seismology and want to become part of a growing community check out our session at the EGU assembly 2024. The integration of seismology within the hydrological community should foster a multidisciplinary approach, enriching research methodologies and enhancing our capacity to predict, manage, and mitigate the impacts of climate change on the (critical) water resources.
We hope to see many of you at our session!
Charlotte, Laura, Yang and Richard
Links and References
Pictures
[1] https://byjus.com/chemistry/water-cycle-process/
[2] Marine A. Denolle, Tarje Nissen-Meyer. Quiet Anthropocene, quiet Earth. Science369, 1299-1300 (2020).
[4] Gaubert-Bastide, T., Garambois, S., Bordes, C., Voisin, C., Oxarango, L., Brito, D., & Roux, P. (2022). High-resolution monitoring of controlled water table variations from dense seismic-noise acquisitions. Water Resources Research, 58, e2021WR030680.
Publications in order of appearance