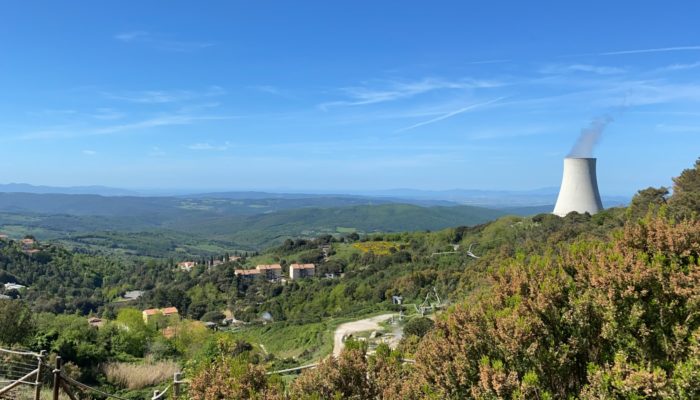
How does a Geothermal system work?
Heat is a form of energy and, strictly speaking, geothermal energy is heat from inside the Earth. The large amount of thermal energy enclosed below the earth’s surface derives in part from its primordial formation process and in part originates from the decay of radioactive isotopes present mainly in the earth’s crust and, secondarily, in the mantle. Thermal flows thus originate towards the earth’s surface and from here, the heat is transferred to the earth’s atmosphere, dispersing in space. On the surface of our planet there are anomalous geothermal areas where the heat flux significantly exceeds the average value, reaching values up to 10 times the average (that is of about 65 mW/m2 in correspondence with continental-type crustal sectors and about 100 mW/m2 in correspondence with the oceanic crust). This occurs mainly at plate margins or in continental areas where lithospheric thinning processes favor asthenosphere ascent. Some well-known geological phenomena (volcanism, geysers, hydrothermal springs, localized gaseous emissions, etc.), represent superficial manifestations of anomalous areas from a geothermal point of view. The energy potential of a geothermal system is generally quantified in terms of enthalpy (state function that provides a measure of the amount of energy that a thermodynamic system can exchange with the environment and is clearly connected to the temperature of the system), and is a function of the geological-structural asset in which the geothermal system is located. Geothermal systems, therefore, are traditionally categorized into two main groups: low enthalpy and high enthalpy geothermal systems. As regards the first type, it is a geothermal system characterized by fluids at temperatures below 90°C, not suitable for the production of electricity, but useful for thermal purposes, i.e. for all applications aimed at the direct use of heat geothermal, from the air conditioning of rooms to the supply of heat in many industrial processes (Fig. 1).
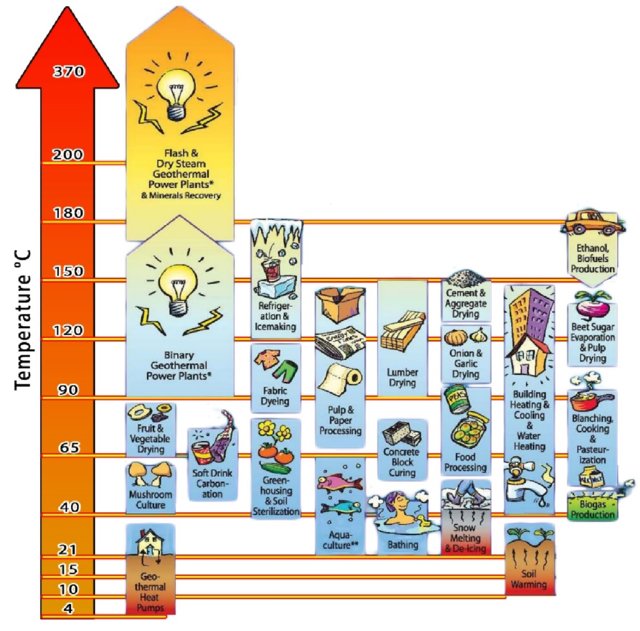
Fig.1 –Lindal diagram: schematically describes the uses of geothermal energy based on the temperature of the system (from the page: https://www.researchgate.net/publication/317388065_Investigation_on_the_potential_of_combined_heat_power_and_metal_extraction_in_Hungary).
Electricity production from geothermal energy
The production of electricity from geothermal sources is possible for medium-high enthalpy sources, starting from about 90°C. In these particular areas, the heat present in the subsoil is used in a substantially analogous way to that produced in traditional fossil fuel or nuclear fission power plants: the extracted steam feeds turbines, connected to alternators that produce electricity.
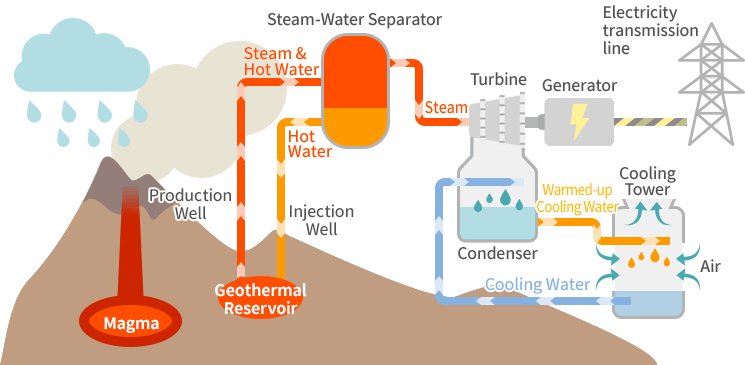
Fig. 2– Simplified diagram of the active dynamics within an industrially exploited hydrothermal geothermal system (from the page: https://www.linkedin.com/pulse/geothermal-energy-jamshed-saleem-member-ieee-member-cigre-/)
The knowledge reached so far regarding the possible geological structure of potential economically cultivable geothermal systems, allow us to distinguish at least 3 types.
- Hydrothermal systems (Fig. 2 and Fig. 3)
- EGS systems (Enhanced Geothermal Systems)
- Unconventional Geothermal Systems (i.e. systems involving the research and exploitation of supercritical fluids (Fig. 3), since they are found in conditions of pressure and temperature higher than those of the critical point of water and have, therefore, have an enormous energy potential)
In order for geothermal energy to be used industrially for the production of electricity, there must be a system, whether natural or the result of engineering works, which is able to convey the thermal energy exchanged in depth to the surface. For this to happen, at least conceptually, the most common functioning geothermal systems must consist of four main elements:
- a thermal source (for example a cooling plutonic body or an active volcano);
- a thermo-vector fluid which transfers the heat to the surface;
- a rocky reservoir in which these fluids circulate and stay;
- an efficient circuit of “recharge” of fluids.
Let’s take a look on how an hydrothermal systems works in detail.
In this tectonic context, the geothermal fluid in circulation consists mainly of meteoric water and possibly of fluids of other origins (magmatic/metamorphic). A necessary condition is that the permeable formations that make up the reservoir are included within levels of impermeable rocks, otherwise the dispersion of the heat-carrying-fluid would be inevitable.
The journey of the meteoric (or juvenile) waters therefore begins following the infiltration on the surface. It deepens into the earth’s crust which is in turn characterized by a geothermal anomaly. This anomaly may be due, as in Larderello (southern Tuscany, Italy), to the presence of a cooling magmatic intrusion (Fig. 3) placed few kilometers below the surface.
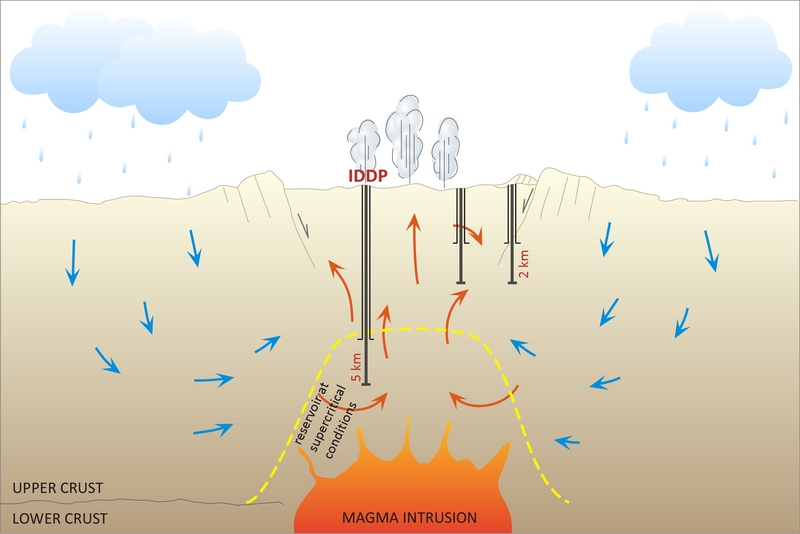
Fig. 3 – Conceptual diagram of wells for geothermal energy production in an simplified hydrothermal context. The possible location of supercritical fluids in the crust is also located, with respect to the cooling intrusion. Picture credit by: G.Ó. Friðleifsson, The Iceland Deep Drilling Project | National Energy Authority of Iceland.
By crossing the permeable rock, the infiltrated fluids undergo heating, become enriched with mineral substances and, rising to the surface, can originate, a vast range of typical geothermal manifestations (hydrothermal springs, travertines, mines located along the structures that allow the transit of fluids, etc.). In such a geological context, the drilling of wells within the reservoir rocks allows the geothermal fluid to be brought to the surface and made available for use in geothermal power plants of various generation. Today it is now common to add wells for the extraction of the fluid to a re-injection well for the geothermal fluid used in order to avoid depletion of the reservoir (binary power plant). For a brief and exhaustive review about the types of geothermal power plants, take a look at the page https://www.linkedin.com/pulse/geothermal-energy-jamshed-saleem-member-ieee-member-cigre-/
Larderello (Tuscany, Italy), the oldest geothermal plant in the world.
In 1911, the world’s first geothermal power plant was built in the Valle del Diavolo (“Devil’s Valley”), southern Tuscany (Italy). This region became the site of a pioneering experiment for the production of energy from geothermal sources in 1904 (take a look at https://www.power-technology.com/features/oldest-geothermal-plant-larderello/ if you are curious!), and the reason is purely geological! In fact, In Tuscany, as well as throughout the Tyrrhenian region, the particular geological structure has provided the ideal conditions for the genesis of an efficient geothermal system. As regards the area of the western side of the Northern Apennines and the Tyrrhenian Sea, it includes that thinned lithospheric and crustal sector (20-26 km) developed as a result of the extensional tectonics which affected the internal sector of the Apennine chain starting from, at least, 14 Ma ago (Liotta e Brogi, 2020). The resulting high flow of heat makes these areas privileged for the industrial use of geothermal resources in Italy, as has been the case for over a century in Tuscany, in the geothermal area of Larderello. The geothermal area of Larderello-Travale and Amiata (Fig. 4), belongs to the so-called “Regione Boracifera“, known since ancient times for its intense, and natural, manifestations related to the rise of geothermal fluids rich in Boron.
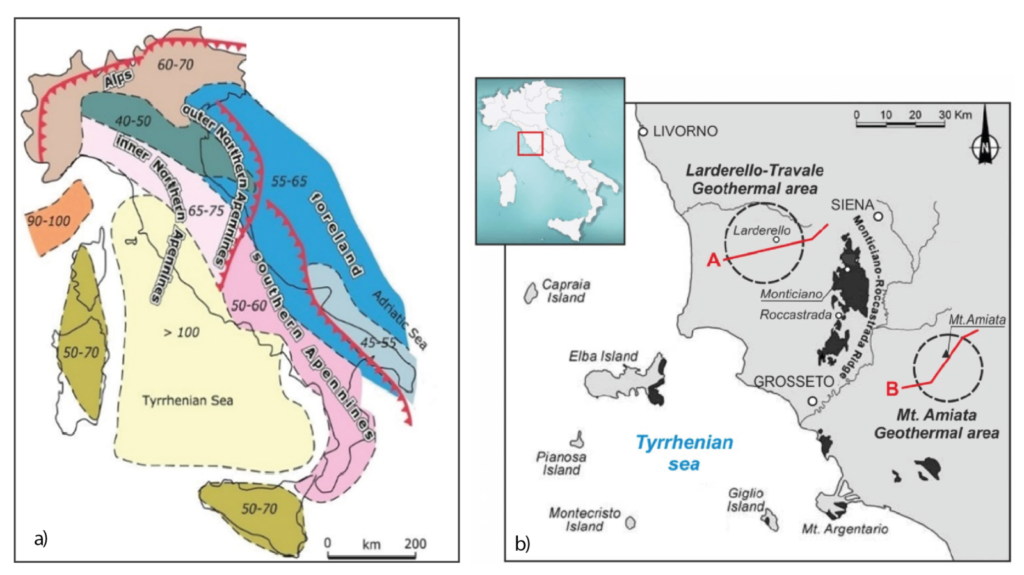
Fig. 4 – a) Schematic Heat flux map (mW/m2) of the Italian territory (modified after Dalla Vedova et al., 2001-2007); b) location of the Larderello and Amiata geothermal areas, with respect to Elba Island (picture from Bellani et al., 2019).
In the present-day active Larderello and Amiata geothermal areas, two reservoirs have been identified: a more superficial one, located in the oldest units of the Tuscan Nappe and a deeper one, still under study (Giannelli et al., 1997; Manzella et al., 1998; Batini et al., 2003; Bertini et al., 2003 ; al., 2006; Santilano et al., 2015; De Franco et al., 2019; Gola et al., 2017; Liotta & Brogi, 2020).
Exploration costs and major drawbacks.
Geothermal energy is a renewable energy resource, inexhaustible on the scale of human use, and sustainable, provided that the use is carried out with a correct engineering approach that is a function of the environmental, tectonic, type of vector fluid and associated chemistry. Compared to fossil fuels, it makes a significant contribution to reducing the emission of greenhouse gases into the atmosphere. However, one of the most debated topics in this regard, together with the impact on the landscape, has to do with the gaseous emissions coming out of the plants. As an example, the up-mentioned geothermal system in Tuscany supplies high temperature geothermal fluids containing various chemical compounds and non-condensable gases (NCGs). Some of these gases, for example hydrogen sulphide (H2S), are separated from the steam and eliminated in steam treatment plants (AMIS filters). The most abundant non-condensable gas in geothermal fluids, CO2, is instead traditionally separated from the steam and released into the atmosphere or used for industrial or agri-food processes. However, it should be emphasized that the nature of the CO2 released by the industrial process itself (the production of energy from geothermal sources) is very different from that produced by fossil fuel-fired thermoelectric plants running on gas, oil or coal (Minissale et al., 1997; Raimondi et al., 2015; Taussi et al., 2022). It is in fact generated naturally in the underlying magmatic systems by degassing of intrusive bodies or by thermo-metamorphic reactions and, as demonstrated by various studies in various areas of the world, including Tuscany, it would still be naturally released from the soil through widespread degassing. Therefore, although in Italy, and above all in Tuscany, there is an enormous latent energy potential, there exists, and cannot be ignored, a certain degree of obstructionism driven by landscape-ecological reasons. Beside this, another obstacle to the development of the use of geothermal resources is in the cost of exploration.
Geothermal drilling cost contribute about 35% of the Total Cost of Geothermal Power Plant Project. As reported by the NQ Geothechnology Company (http://www.newquest-geotechnology.com) a study conducted by Directorate of Geothermal, General Directorate of New and Renewable Energy and Conservation Energy (EBTKE), based on data 2018 and 2019, resulting drilling costs are in the range of $ 3M – $ 12M per well with an average drilling cost of $ 2,500-3,800/m. Given the huge construction costs, ensuring the success of a well, in terms of productivity and longevity, is essential. But how to know where the productivity of the system is maximized, and consequently, where is better to drill? Few people consider that the answer to this question is partially guaranteed by the study of fossil geothermal systems.
How the study of exhumed geothermal systems can reduce risks related to direct exploration? An example from the Elba Island, Tuscan Archipelago (Italy)
Since about 14 Ma extensional tectonics affected the Inner sector of the Northern Apennines and triggered the emplacement of magmatic bodies at shallow crustal levels (< 6-7 km). This processes, which have the main influence on the up-mentioned thermal anomaly characterizing the whole Thyrrhenian area (Fig. 4), migrated eastward, behind the Apennine compressive front, making the tectonic conditions having brought to magmatism remain stable through time (Carmignani et al., 1994). The presence of exhumed plutonic bodies pertaining to the Tuscan Magmatic Province (i.e. both exposed plutons from Elba Island and the cooling granite from the Larderello area pertaining to this magmatic province; Fig. 3) offer an exceptional opportunity to construct a conceptual model on the relationships between geological structures and geothermal fluid flow (i.e. Raimondi et al., 2015; Liotta et al., 2021 and references therein). More practically, fracture systems, and the related paleo-stress field, tell us about the directions of enhanced secondary permeability in the brittle crust. So, if a geometrical coherence of structures and stress field between the fossil geothermal system and the active area under exploration is found, it is possible, by analogy, to obtain key parameters to optimize the geothermal exploration, being in possession of a key information (that, obviously, should be coupled to borehole logs, geophysics and geochemistry, if available) for the research of the potentially more productive areas. Another powerful methodology which can greatly improve the construction of such a conceptual model, and then improve geothermal exploration, is the study of paleo-geothermal fluids trapped in hydrothermal veins exposed in presence of exhumed magmatic systems. In fact, the study of fluid inclusions provides information about the temperature and pressure at which fluids where trapped in the minerals, as well as information about the density, viscosity, and chemistry of the fluid circulating in the hydrothermal system, being all information which are also very useful from an engineering point of view. For further insights into the topic, take a look here! (https://www.mdpi.com/1996-1073/14/4/933)!
References
Batini F., Brogi A., Lazzarotto A., Liotta D., and Pandeli E. (2003) – Geological features of Larderello-Travale and Mt.Amiata geothermal areas (southern Tuscany, Italy). Episodes · September 2003.
Bertini G., Casini M., Gianelli G., and Pandeli E., (2006) – “Geological structure of a long-living geothermal system, Larderello, Italy,” Terra Nova, vol. 18, no. 3, pp. 163–169, 2006.
Carmignani, L., F. A. Decandia, L. Disperati, P. L. Fantozzi, A. Lazzarotto, A. Liotta, and G. Oggiano (1995) – Relationships between the Tertiary structural evolution of the Sardinia-Corsica-Provencal Domain and the northern Apennines, Terra Nova, 7, 128 – 137.
Della Vedova B., Vecellio C., Bellani S., Tinivella U. (2007) – Thermal modelling of the Larderello geothermal field (Tuscany, Italy). Int J Earth Sci (GeolRundsch)DOI 10.1007/s00531-007-0249-0.
Gianelli G., Manzella A., and Puxeddu M. (1997) – “Crustal models of the geothermal areas of southern Tuscany (Italy),” Tectonophysics, vol. 281, no. 3-4, pp. 221–239.
Gola G., Bertini G., Bonini M. et al., (2017) – Data integration and conceptual modelling of the Larderello geothermal area, Italy. Project: Integrated Methods for Advanced Geothermal Exploration (IMAGE). September 2017Energy Procedia 125:300-309 DOI: 10.1016/j.egypro.2017.08.201
Liotta D., Brogi A. (2020) – Pliocene-Quaternary fault kinematics in the Larderello geothermal area (Italy): Insights for the interpretation of the present stress field. January 2020Geothermics 83:101714. DOI: 10.1016/j.geothermics.2019.101714. Project: IMAGE FP7.
Liotta, D.; Brogi, A.; Ruggieri, G.; Zucchi, M. Fossil vs. Active Geothermal Systems: A Field and Laboratory Method to Disclose the Relationships between Geothermal Fluid Flow and Geological Structures at Depth. Energies 2021, 14, 933. https://doi.org/10.3390/en14040933
Manzella A., Ruggieri G., Gianelli G., and Puxeddu M. (1998) – “Plutonic-geothermal systems of southern Tuscany: a review of the crustal models,” Memorie Società Geologica Italiana, vol. 52, pp. 283–294.
Minissale A., Evans C.W, Magro G., Vaselli O., (1997) – Multiple source components in gas manifestations from north-central Italy. Chemical Geology 142 (1997) 175 – 192
Rimondi V., Fregola R.A. , Ruggieri G. , Zucchi M. , Chiarantini L. , Orlando A. , Brogi A. & Liotta D. (2015) – Investigating fossil geothermal systems: the case of Elba Island (Tuscany, Italy).
Santilano, A., Manzella, A., Gianelli, G., Donato, A., Gola, G., Nardini, I., Trumpy, E., Botteghi, S. (2015a) – Convective, Intrusive Geothermal Plays: what about tectonics? Geothermal Energy Science, 3, 51-59. doi:10.5194/gtes-3-51-2015
Taussi M., Brogi A., Liotta D., Nisi B., Perrini M., Vaselli O., Zambrano M., Zucchi M., (2022) – CO2 and heat energy transport by enhanced fracture permeability in the Monterotondo Marittimo-Sasso Pisano transfer fault system (Larderello Goethermal Field, Italy). Geothermics 105(1–2):102531. DOI:10.1016/j.geothermics.2022.102531