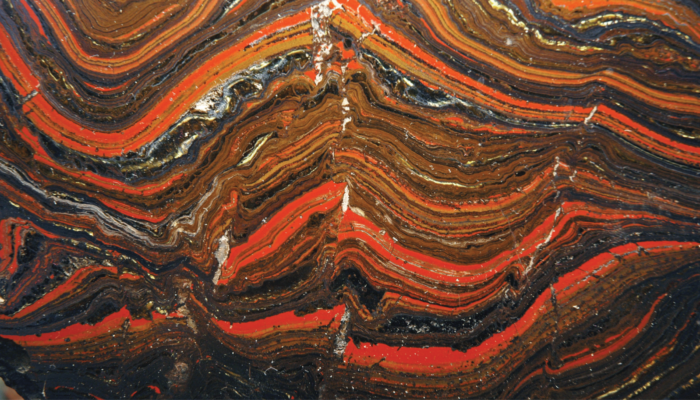
In the recent past, a lot of effort has been directed toward evaluating the consequences of the rapidly rising global temperatures. Among them, one very alarming consequence is ocean acidification (Fauville et al, 2012; Gatusso et al, 2015).
Ocean acidification is the direct result of atmospheric CO2 increase caused in part by anthropogenic activities such as burning fossil fuels for energy activities (Fauville et al, 2012); one third of CO2 is absorbed by the ocean water, where it becomes partially converted into carbonic acid – H2CO3 (Fig. 1; Sosdian et al, 2018). From here a reaction chain leading to a lower pH of the ocean is initiated: carbonic acid dissociates to bicarbonate ions (), which continue to dissociate to carbonate ions () (Albright et al, 2010). During this process, H+ ions are released into the water, which lower the ocean pH causing more acidic (although with pH > 7) conditions (Mitchell et al., 2009). The presence of excess H+ then induces the enhanced production of bicarbonate ions through the reaction of H+ with the carbonate ions in the water (Fig. 1). However, carbonate ions are an essential component for the formation of calcium carbonate (CaCO3) – the fundamental building block of many marine organisms (e.g. corals or foraminifera). If seawater becomes undersaturated in , this can additionally induce the dissolution of CaCO3 exoskeletons, endangering marine organisms that rely on their protective shells (Barker & Ridgwell, 2012).
The effects of global warming in the past can be seen in sedimentary rock structures. For example, warmer climatic conditions are associated with prolonged chemical weathering, which is seen in the removal of labile (i.e. easily decomposable) minerals from sands and the production of aluminum – and iron-rich (i.e. lateritic) clays, while colder conditions are responsible for the relative abundance of mineral phases such as ikaite (CaCO3–6H2O), mirabilite (Na2SO4–10H2O), natron (Na2CO3–10H2O) and hydro-halite (NaCl–2H2O) (Smoot et al, 2009).
A more challenging question, however, is whether sedimentary rocks can provide a record of the actual pH of the water at the time of their deposition. The answer is yes. One possible method for doing so includes the isotopic ratios of boron in sediments, which represent the corresponding boron isotope ratios directly linked to the water’s pH and CO2 levels.
In seawater, boron is mostly found either as boric acid (H3BO3) or as borate (BO3-3), the ratio of which depends on the pH of the water. Boric acid has been shown to “prefer” the heavier boron-11 isotope as opposed to the lighter boron-10 isotope (Carrano et al, 2009). Corals and shell-forming organisms contain borate (BO3-3), with a boron-11 concentration dependent on the presence of boric acid (Sosdian et al, 2018). In other words, when the ocean pH is high and less boric acid is available, concentrations of the boron-11 isotope increase in the water and with that also in marine biogenic carbonates. Or on the other side, when the boric acid becomes more abundant due to lower ocean pH, the boron-11 isotope is less frequently implemented in marine biogenic carbonates.
These isotope records preserved in marine carbonate sediments cover the last 500 million years, providing an unparalleled geochemical archive of the past climate. Therefore, enabling scientists to estimate the pH of seawater and the climate conditions from the distant past (Jacob et al, 2017). For instance, Trilobatus trilobus is widely used for such geochemical and paleoclimatic reconstructions since it has survived both the Neogene and Quaternary periods with little genetic changes to its shell composition. The study of their shells strongly suggests that warmer climatic phases correspond with lower pH and that in the past such large acidification processes are associated with mass extinction events. For example, in the early and middle Miocene, fossil records show a substantial, though not total, extinction of corals and mollusks related to lower pH, probably induced by increased temperatures (Sosdian et al, 2018).
However, one must keep in mind that the climate fluctuations in the past occurred much slower than those in the present, leaving marine organisms with enough time to adapt to these changes. Meanwhile, it has been predicted that by 2100 our climate will reach pH levels unprecedented during the past 14 million years, coupled with an increasingly large rate of change over such a relatively short period of time (Sosdian et al, 2018).
While there is uncertainty about all the possible effects these climatic circumstances could have on the global marine ecosystems, there are already some visible consequences on the horizon. For instance, experiments with oysters have shown that shell synthesis is slowed down and the shells themselves become weaker when the pH is decreased by adding CO2 (Fig. 1, Speights et al, 2017). As a result, population numbers of oysters, as well as other shell-bound organisms, are likely to decline. Another problem is the bleaching of corals in response to the rise in water temperature, which can disrupt entire ecosystems since they are home to approximately 25% of all marine species (Hughes et al, 2017; Rafferty, John P., 2022).
In the past, the processes of extreme global warming (or cooling) were counteracted by our Earth’s natural adaptation mechanisms stimulating (or slowing down) processes such as chemical rock weathering (for example the dissolution of terrestrial limestone in presence of acidic rain) to maintain an equilibrium. The problem is that the rapid pace of human activity threatens to bypass these natural buffer mechanisms, which could result in irreversible damage. It is, therefore, crucial to understand the extent of these planetary boundaries by analyzing how far the climate fluctuated in the past and the corresponding consequences of such events. For these purposes, sedimentary formations are immensely valuable because of the enormous time scale they span and the detailed record they hold as seen in the example of boron isotope ratios.
References: Albright, Rebecca, et al. “Ocean Acidification Compromises Recruitment Success of the Threatened Caribbean Coral Acropora Palmata.” Proceedings of the National Academy of Sciences, vol. 107, no. 47, 2010, pp. 20400–20404., https://doi.org/10.1073/pnas.1007273107. Barker, S. & Ridgwell, A. (2012) Ocean Acidification. Nature Education Knowledge 3(10):21 Bolli, H. M., Saunders, K. Perch-Nielsen, editors, 1985: Plankton stratigraphy Cambridge University Press 599 pp. Plate 20, Fig 14 Carrano C.J et al: “Boron and Marine Life: A New Look at an Enigmatic Bioelement”. Mar Biotechnol 11, 431 (2009). https://doi.org/10.1007/s10126-009-9191-4 Fauville, G., et al. “Impact of Ocean Acidification on Marine Ecosystems: Educational Challenges and Innovations.” Marine Biology, vol. 160, no. 8, 2012, pp. 1863–1874., https://doi.org/10.1007/s00227-012-1943-4. Gattuso, J-P., et al. "Contrasting futures for ocean and society from different anthropogenic CO2 emissions scenarios." Science 349.6243 (2015). Hughes et al: “Global warming and recurrent mass bleaching of corals”. 2017, Nature, 543 (7645). pp. 373-377. ISSN 0028-0836 Jacob D.E. et al: “Planktic foraminifera form their shells via metastable carbonate phases”. Nat Commun 8, 1265 (2017). https://doi.org/10.1038/s41467-017-00955-0 Mitchell Mark J., et al: “A model of carbon dioxide dissolution and mineral carbonation kinetics.” Proc. R. Soc. A.4661265–1290 http://doi.org/10.1098/rspa.2009.0349 Rafferty, John P."coral bleaching". Encyclopedia Britannica, 25 Mar. 2022, https://www.britannica.com/science/coral-bleaching. S.M. Sosdian, et al.: “Constraining the evolution of Neogene Ocean carbonate chemistry using the boron isotope pH proxy,” Earth and Planetary Science Letters, 2018, Volume 498, Pages 362-376. Smoot J.P.: “Sedimentary Indicators of Climate Change”, 2009, In: Gornitz V. (eds) Encyclopedia of Paleoclimatology and Ancient Environments. Encyclopedia of Earth Sciences Series. Springer, Dordrecht. https://doi.org/10.1007/978-1-4020-4411-3_210 Speights, CJ, Silliman et al: “The effects of elevated temperature and dissolved ρCO2 on a marine foundation species”. Ecol Evol. 2017; 7: 3808– 3814. https://doi.org/10.1002/ece3.2969