Most of what we know about dinosaurs comes from their skeletal remains. Rarely, we get tiny glimpses into their soft tissue anatomy through skin impressions and even rarer, preserved tissue fragments, mummified over time, and their ecology and life habits through combining interpretation of this from what we can glean from trace fossils (footprints, poop, etc.). Palaeontologists are also taking the first steps to digitally reconstructing their muscular systems through looking at muscle attachment points on bones and comparing this with their living archosaur relatives, crocodiles and birds. But what if we could actually peer inside their skulls to look at their brains? Brains, unsurprisingly, are not preserved in the fossil record. This is due to two, equally scientifically valid points – brains are nutritious, and when a dinosaur dies, their brains are usually scavenged by other carnivores so that they can assimilate the brain-host’s knowledge (and their hearts, for courage)*, and secondly, soft tissue does not readily preserve under normal taphonomic conditions, and only exceptionally rarely under the right conditions, which are typically deep marine anoxic environments (not something any known dinosaur is yet known to have inhabited).
What we do infrequently find though, are dinosaur skulls preserved intact in three-dimensions, with the hollow neural centre of the skull, which preserves a mould of the braincase as it would have been when the organism was still alive. There are two main methods for looking at the anatomy of this part of the skull: pump it full of some rubbery substance like latex, let it set, then pull it out (like an Egyptian mummy) or break the skull open to retrieve it (almost as fun as a piñata); or use a CT-scanner. Computed tomography uses x-rays to identify differences in density of a scanned object, providing a three dimensional visualisation of this. The braincase, being hollow, will naturally come up as a different region to the substantially denser surrounding fossilised bone. What you get, is a three-dimensional representation of the neuroanatomy of a braincase!
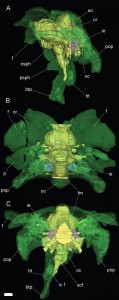
Braincase of Bonatitan, digitally reconstructed (green) with cranial endocast (yellow). A = left lateral, B – dorsal (from above), C = Posterior (from behind). Scale bar = 10 mm. Anatomical Abbreviations: bt, basal tuber; btp, basipterygoid process; f, frontal; fm, foramen magnum; ic.f, internal carotid foramen; ie, inner ear; oc, occipital condyle; osph, orbitosphenoid; or, orbital rim; p, parietal; pop, paroccipital process; psph, parasphenoid; scf, subcondylar foramen; so, supraoccipital (Copyright: Calabajal 2012; click for larger image)
The most recent application of this was to a group of sauropod dinosaurs known as titanosaurids. Three taxa from Argentina, Bonatitan, Antarctosaurus, and a third un-named titanosaur were CT-scanned, revealing their neuroanatomy in quite high resolution, which is a pretty cool application of a medical technique to the fossil record. These compliment a series of cranial endocasts of a host of other sauropod taxa. What these endocasts represent, is not a reflection of the shape of the brain exactly, but the morphology of the endocranial space which includes the brain and also other structures such as meninges (system of membranes enveloping the central nervous system) and venous sinuses (channels found within layers of the brain), as well as digital representations of the inner ear.
What the study found is that the sauropod endocranial structure is globose (awesome word) and transversely wide, and differs from derived maniraptoran dinosaurs (the ancestors and close relatives of birds) in lacking meningeal vessel traces. Titanosaurids also appear to have had a tall dorsum sellae, the function of which is still uncertain but may suggest an enlarged pituitary gland, which may also be related in some way to body size, but also is related to developmental and reproductive function in other archosaurs, so the precise role in titanosaurs is still unclear.
In the analysed titanosaurids, the floccular recess was also absent. Comparative analysis of this with the neuroscience of extant species it appears that this corresponds with co-ordinating inputs from the periphery and vestibular apparatus to enhance the vestibu-occular reflexes (eye co-ordination), which also ties into the reduction of the semi-circular canals in the inner ear, suggesting that titanosaurids had a decreased range of head movements. Within saurischians (theropods and sauropodomorphs), it is likely that this is intimately tied to the degree of bipedality.
So yeah, a pretty cool study combining palaeontology, medical technology, and neuroscience, giving early footsteps into understanding the neural systems of this extinct but jaw-droppingly awesome group of dinosaurs.
*This might be a lie.
Reference:
A. P. Carabajal (2012) Neuroanatomy of titanosaurid dinosaurs from the Upper Cretaceous of Patagonia, with comments on endocranial variability within Sauropoda, The Anatomical Record, 295, 2141-2156