The short lifetime of aerosol particles in the atmosphere means that they tend to be found concentrated in regions close to where they were formed. As they only last for days to weeks in the atmosphere, they don’t travel too far as the Earth’s winds aren’t able to fully mix the air over such a short period.
This short lifetime should also mean that any changes in the emissions of aerosol particles and their gas-phase building blocks will show up quickly when we measure their concentrations in the atmosphere. This contrasts with greenhouse gases, which typically have much-longer lifetimes (decades to centuries), so their concentrations remain much further into the future (even if their emissions were ceased completely).
Below is a collection of images of the global aerosol view from 2001 to 2012 from the Multi-angle Imaging SpectroRadiometer (MISR) on board NASA’s TERRA satellite. Each map is a single year, with the year noted to the bottom right of the image. The maps show the Aerosol Optical Depth (AOD), which is a measure of the amount of aerosol within the atmosphere. As the measurements are made from space, the instrument measures the aerosol in a vertical column below the satellite. Terra orbits the Earth continuously and gradually builds up a picture of the Earth below; MISR itself will typically build up a global picture over a couple of weeks.
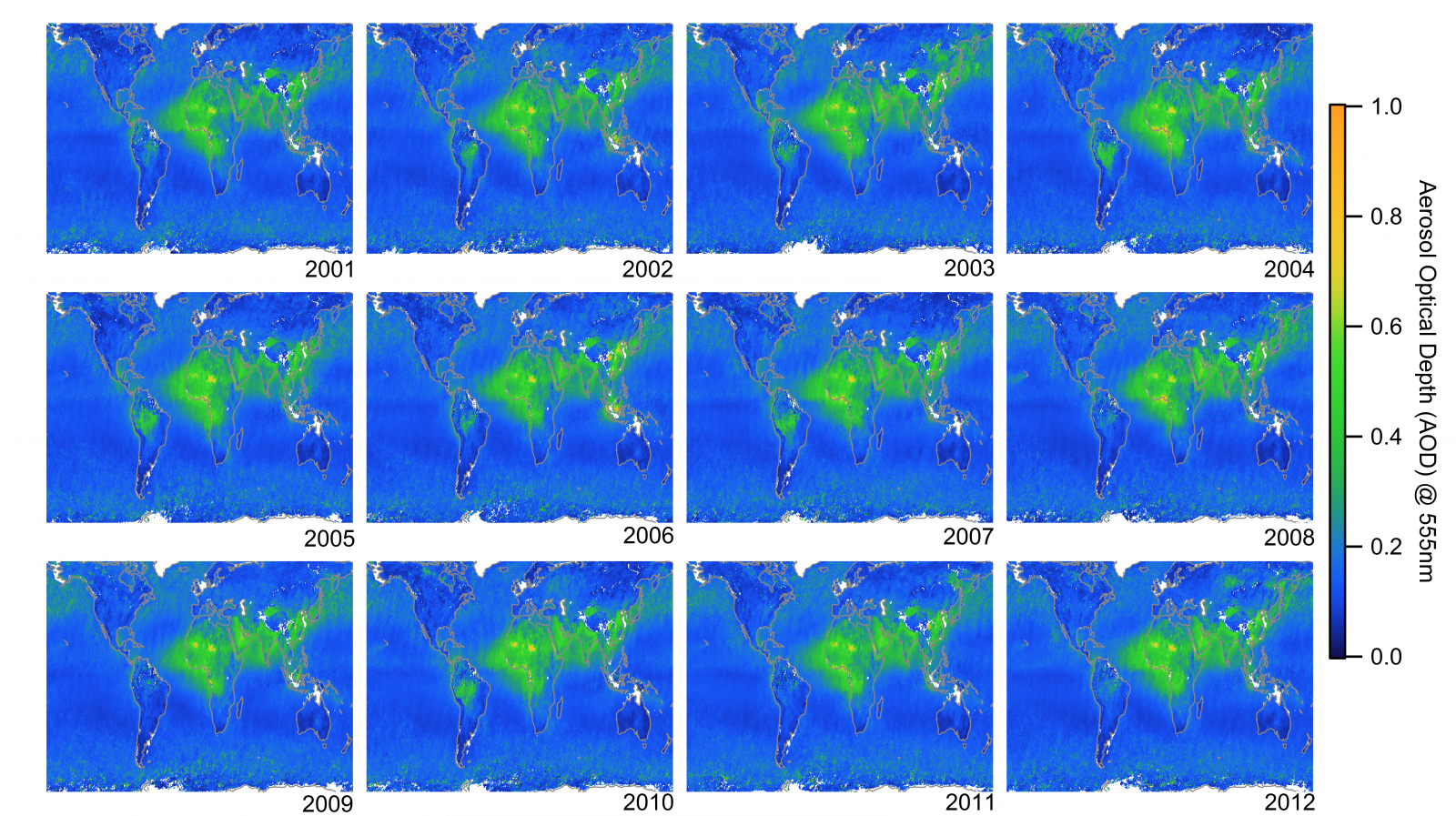
Global view of the Aerosol Optical Depth (AOD) from 2001-2012 observed by the Multi-angle Imaging SpectroRadiometer (MISR) on board NASA’s TERRA satellite. White spaces indicate there is no data. High latitudes are excluded as the satellite retrievals don’t work over highly-reflective surfaces (snow/ice). Click on the image for a larger view.
The most persistent feature is the large expanse of aerosol over Africa, which also extends into the Atlantic Ocean. Over North Africa, much of this aerosol is a result of the wind whipping up a storm of Saharan dust, which can then be blown over large distances. Sometimes, the dust even makes its way across the whole Atlantic Ocean and lands in North or South America. Further south, frequent burning occurs to clear savannah areas in Southern Africa, which creates large amounts of smoke.
The Arabian Peninsula, India and South-East Asia (particularly China) are other regions where aerosol is prevalent. Similarly to the Sahara, Saudi Arabia is prone to large dust storms, while India and China have undergone significant economic development recently, which has led to increased burning of fossil fuels. South America also sees a large build up of aerosol over the Amazon Rainforest due to deforestation fires, although the amount of aerosol varies more from year-to-year.
Europe, North America and Australia display relatively low levels of aerosol compared to these other regions. Bear in mind that these are annual averages, so they will miss intense and short-lived pollution events, which do still affect these regions.
The atmosphere above the Earth’s oceans is generally quite clean as far as aerosol is concerned, as the major sources of aerosol are far away. There are deviations from this general observation though, for example, aerosol is transported across the Pacific Ocean from Asia to North America. This can be seen in the top right of each image (as well as in the top left at times). The strong winds in the Southern Ocean also see elevated aerosol amounts, as the wind helps to produce sea spray aerosol.
Below is a map showing the difference between the last four years shown above (2009-2012) and the first four years (2001-2004) in order to highlight changes in aerosol over this period. Overall, there hasn’t been a great change in the global amount of aerosol; Dan Murphy, in a paper in Nature Geoscience (pay walled, sorry), showed that the level of aerosol hasn’t changed much over this period but that there have been regional changes. This is in agreement with several other papers using similar and/or the same measurements (some non-pay walled papers here and here). From a statistical point of view, the trend in global aerosol is negligible and weakly positive i.e. aerosol levels have increased since the turn of the century but the increase isn’t statistically significant.
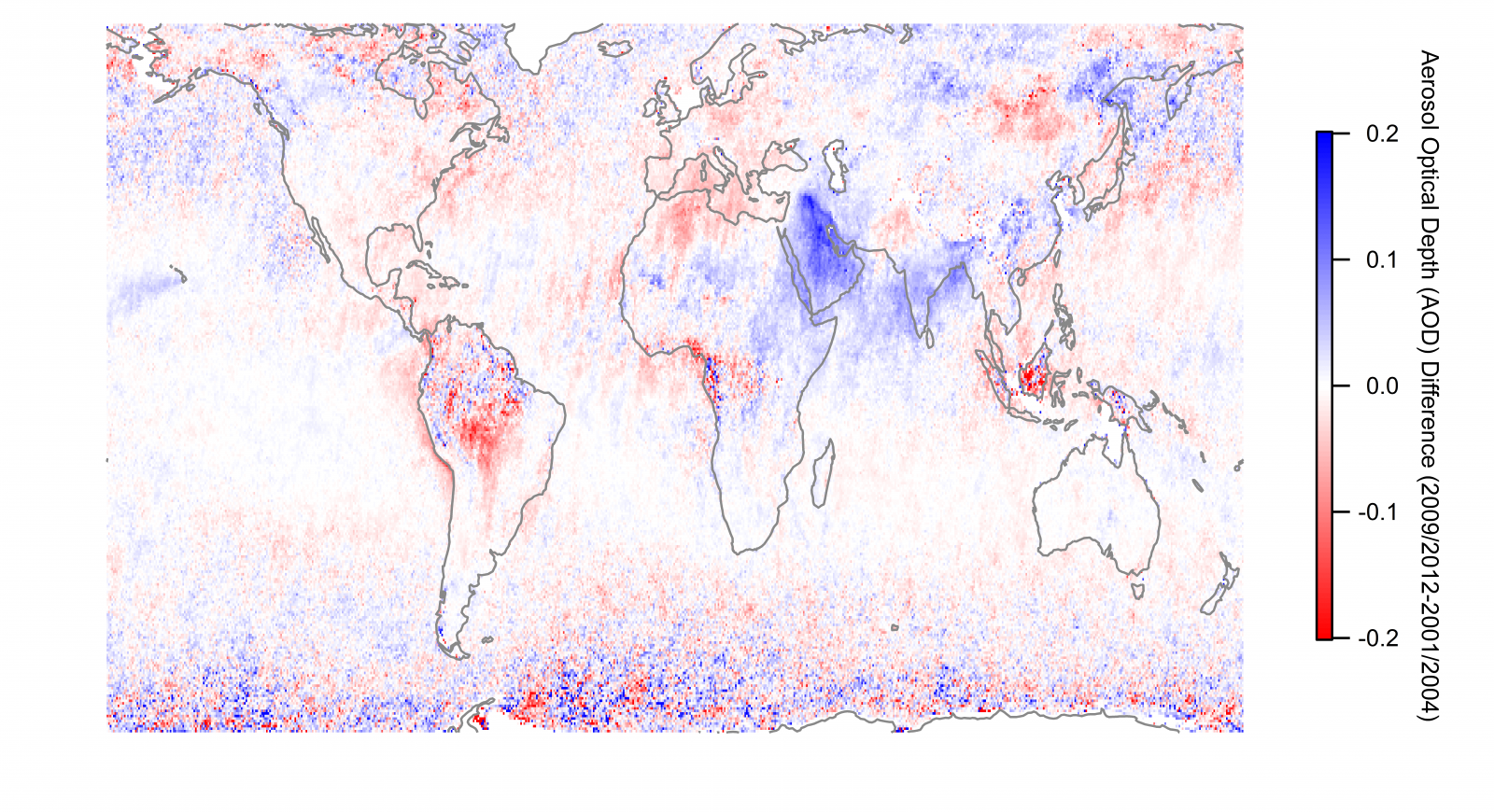
Difference in Aerosol Optical Depth (AOD) from 2009-2012 to 2001-2004. Data source is the same as above. Blue colours indicate an increase in aerosol, while red colours show a decrease.
The strongest increases have been over the Arabian Peninsula and India. These increases are likely a consequence of natural causes in the case of Saudi Arabia via increased dust emissions and transport, whereas India is likely a consequence of increased burning of fossil fuels. South America is probably the most notable region as far as aerosol decline is concerned; in recent years, deforestation has decreased compared to the early 2000s combined with some wetter years over the Amazon. Significant burning does still occur though, with 2010 being a notable year where drought conditions meant that a very large amount of smoke built up over the Amazon during the biomass burning season. There have also been declines in aerosol over Europe and the North Eastern USA, which are likely due to decreased emissions of sulphur dioxide from coal power stations.
The upshot of these trends is that since the year 2000, aerosol particles have undergone relatively minor changes on the global scale. The question going forward is whether this will continue and what impact will such changes have on our climate. Thus far, the declines in Europe and North America have been offset by increases in Asia, although the climate-relevant properties of the aerosol may not be the same. Furthermore, much of the decline in aerosol has been driven by reductions in sulphate aerosol but other species, such as secondary organic aerosol and ammonium nitrate, may become comparatively more important. These species are often missing or poorly represented in climate models, which has been suggested as an explanation for the recent pause/hiatus/slowdown in global mean surface temperature.
This is clearly a major issue in climate science currently and the role of aerosol is unclear. I’ll be writing more on this topic in the future, so watch this space!
—————————————————————————————————————————————————————–
Header image: Dust and Clouds Dance Over the Sahara, published by NASA Earth Observatory. The photograph was taken by astronaut Alex Gerst on September 8, 2014, from the International Space Station.
MISR analyses and visualizations used in this blog post were produced with the Giovanni online data system, developed and maintained by the NASA GES DISC.