Explaining the Mysterious Plethora of Short Magnetic Field Reversals Observed by Parker Solar Probe and their Relation to the Origin of Solar Wind.
In Switchbacks Explained: Super-Parker Fields – the Other Side of the Sub-Parker Spiral, N. A. Schwadron and D. J. McComas provide a simple geometric explanation for the source of “switchbacks” and associated large and one-sided transverse flows in the solar wind observed by Parker Solar Probe as it ventured close to the Sun. The mystery of the predominance of switchbacks is a fundamental clue to the origin of the solar wind and the structure of the magnetic field that fills our heliosphere.
The solar wind rapidly accelerates in the corona at 2 – 5 solar radii and then becomes super-Alfvenic at 10 – 20 solar radii (Katsikas et al. 2010; Goelzer et al. 2014). It is this latter transition where the solar wind ram pressure becomes dominant, overcoming both the magnetic and thermal pressure. The processes that transfer energy and dissipate this energy to heat the corona and power the solar wind remain critical questions in heliophysics and astrophysics, and are at the heart of the scientific motivations for the Parker Solar Probe (PSP) mission (McComas et al. 2007; Fox et al. 2016). On PSP, the solar wind is observed by the Solar Wind Electrons Alphas and Protons Investigation (SWEAP) (Kasper et al. 2016) and the magnetic field by the Electromagnetic Fields Investigation (FIELDS) (Bale et al. 2016). The Integrated Science Investigation of the Sun (ISOIS ) instrument suite (McComas et al. 2016) provides comprehensive measurements of energetic particles.
One of the key discoveries from PSP is the observation of thousands of intervals (duration from seconds to tens of minutes) where the speed of the solar wind suddenly jumps and includes a large, one-sided transverse flow, while simultaneously the magnetic field orientation rotates through large angles, before returning to roughly the prior solar wind conditions. The observed switchbacks (radial magnetic field reversals) are associated with the change in magnetic field direction and velocity spikes associated with the sharp increase in solar wind speed (Bale et al. 2019; Kasper et al. 2019; de Wit et al. 2020; Horbury et al. 2020; Mozer et al. 2020; Rouillard et al. 2020; Tenerani et al. 2020).
Magnetic switchbacks have been studied extensively in fast wind from coronal holes (e.g., Kahler et al. 1996) at 1 au, and beyond 1 au with Ulysses (e.g., Balogh et al. 1999; Yamauchi et al. 2004; Neugebauer & Goldstein 2013). Observations of switchbacks have also been made inside 1 au with Helios (Borovsky 2016; Horbury et al. 2018) prior to the observations by PSP. Strong magnetic field deviations from the Parker Spiral are observed where there are local increases in the radial solar wind speed (Michel 1967), and associated with one-sided or pulsed Alfvenic fluctuations (Gosling et al. 2009, 2011). The one-sided nature of switchbacks is especially clear in PSP observations.
The transverse flows observed by PSP far exceed those in the now classic Weber-Davis model (Weber & Davis 1967) where the lower corona is taken to rotate rigidly with the Sun. To this point Kasper et al. (2019) state: “The large rotational velocities measured .. exceed the value in the axisymmetric Weber-Davis model .. by more than an order of magnitude.” The one-sided transverse flows are a key observable from PSP that any switchback theory must also explain. The Schwadron and McComas (2021) paper makes a fundamental connection between the recent observations made by PSP and the observations of previous missions (Ulysses, ACE, and STEREO) showing large deviations of the heliospheric magnetic field from the traditional Parker Spiral.
The first observations that the heliospheric magnetic field deviates significantly and systematically from the Parker Spiral came from observations of the magnetic field in “rarefaction regions” (Murphy et al., 2002). Large-scale rarefaction regions in the solar wind are created where the fast wind out runs slower wind behind. These structures typically form in so-called Co-rotating Interaction Regions where fast and slow wind interact. In the growing region between the fast and slow solar wind flows, the density drops significantly and the solar wind speed transitions steadily from fast to slow wind speeds. Schwadron (2002) showed that differential motion at the Sun’s surface drags the footpoints of the magnetic field lines from the source of fast wind into the source of slow wind. This results in magnetic field lines that are connected from fast wind into slow wind. Therefore, as the fast wind outruns the slower wind behind it, the magnetic field becomes increasingly stretched out, and develops large deviations in the radial direction. A similar effect was reported by Gosling and Skoug (2002) but associated with abrupt temporal variations in the solar wind rather than a systematic boundary between fast and slower wind. Figure 1 is taken from Schwadron et al. (2005) and shows the large difference between a standard Parker Spiral (panel a) and a “Sub-Parker Spiral” (panel b) that has its magnetic field stretched between fast and slow wind. The more radial, Sub-Parker Spiral structure of the heliospheric magnetic field was observed by the Ulysses, ACE, and STEREO missions.
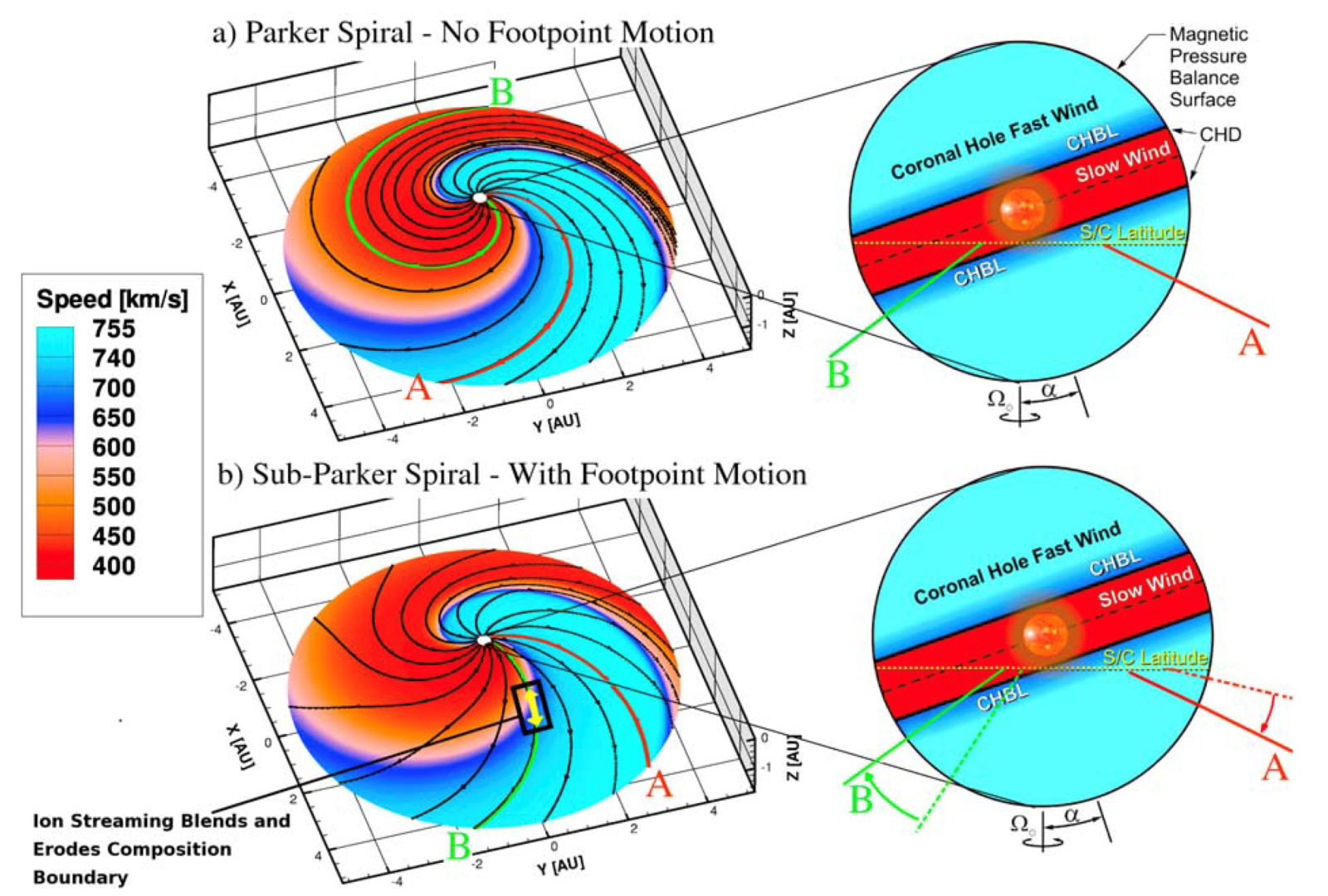
Figure 1. Configuration of magnetic fields in a Co-Rotating Interaction Region (CIR) (a) without and (b) with footpoint motions at the Sun. Differential rotation at the Sun of magnetic field footpoints creates a magnetic connection across the interface between fast and slow wind, causing magnetic field lines (black lines) to cross these interfaces. The coloring shows the speed of solar wind. In the right cutouts we illustrate the general solar minimum configuration that gives rise to a Co-rotating Interaction Region. We illustrate several magnetc field lines, labeled A and B, mapped through the inner heliosphere. Also shown are the Sun’s rotation axis and the offset of the center of the coronal hole by an angle. With footpoint motion, field lines are connected across the stream interface. Taken from Schwadron et al., 2005.
The concept of the Sub-Parker Spiral is also illustrated in Figure 2 (top panel). The magnetic field, stretched between faster solar wind flow and slower solar wind becomes increasingly radial with distance from the Sun. The basic prediction in this case is the association between rarefaction and the Sub-Parker Spiral magnetic field, which has a stronger radial component than the Parker Spiral. A recent study by Schwadron et al. (2020) found that the Sub-Parker Spiral provides relatively short magnetic field line connections from the PSP spacecraft to the compressions and shocks surrounding Co-rotating Interaction Regions in the inner heliosphere (at ∼0.7 – 10 au). These shorter magnetic field line connections are essential in explaining the persistence of energetic particles (from ∼ 100 keV to > MeV) observed by ISOIS up to ∼1 week after the passage of a stream interface between fast and slow wind.
When magnetic field footpoints move from the source of slow wind into faster wind, magnetic field switchbacks are formed as the faster wind overtakes adjacent slower wind flows and literally bends the magnetic field back on itself. This magnetic configuration, or Super-Parker Spiral, illustrated in the bottom panel of Figure 2 is the opposite of the Sub-Parker Spiral magnetic structure. The fundamental difference between the Sub-Parker Spiral and the Super-Parker Spiral is the direction of footpoint motion: footpoint motion from the source of slow wind into faster wind creates the Super-Parker Spiral; the opposite orientation of footpoint motion from the source of fast wind into the source of slow wind creates the Sub-Parker Spiral. The source of faster flow can come from coronal holes, transients associated with interchange reconnection, loop sources, plumes, spicules or macoscipules. These sources of solar wind variability have been noted as potential causes of transient jets or speed variations in the solar wind.
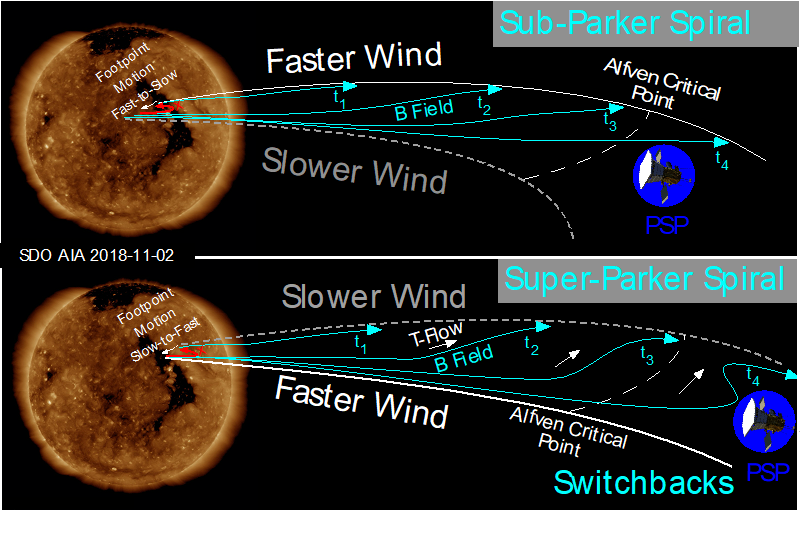
Figure 2. The Sub-Parker Spiral (top panel) and the Super-Parker Spiral (bottom panel) result from footpoint motion between source regions of fast and slow solar wind. In the case of the Sub-Parker Spiral, footpoint motion from the source of fast to slow wind creates a field line connection that gets straightened as fast wind drags out magnetic field lines more quickly than the slow wind. The Sub-Parker Spiral is associated with magnetic field lines with larger radial components than the Parker Spiral. When footpoint motion is reversed (bottom panel), and footpoints move from the source of slow into fast wind, then the wind shear kinks the magnetic field. In this case, the faster wind moves along the magnetic field below the Alfven critical point, forming compressions and tangential flows and develops into a switchback above the Alfven critical point. From Schwadron and McComas, 2021.
There is a critical boundary close to the Sun called the Alfven critical point, thought to be between 10 and 20 solar radii. Below the Alfven critical point, the magnetic field is strong enough so that the tension of the magnetic field is able to resist the flow of the solar wind. Above the Alfven critical point, the situation reverses, and the ram pressure of the solar wind dominates over the tension in the magnetic field. Below the Alfven critical point, the large tension of magnetic field lines enables them to behave like steel rods and resist bending. This forces the plasma flows to move along the magnetic field below the Alfven critical point. Therefore, faster wind overtakes slower wind below the Alfven critical point, forming plasma compression and transverse flow along the field. In other words, below the Alfven critical point, one-sided transverse field-aligned flows develop along the Super-Parker Spiral.
Above the Alfven critical point, the solar wind flow dominates over the magnetic field tension, and the magnetic field lines bend like flexible ropes in the solar wind flow. As a result, along the Super-Parker Spiral magnetic field, the field structure contracts between adjacent solar wind flows, and the radial field component of the magnetic field decreases in magnitude with distance from the Sun, eventually reversing into a switchback. Imagine a long rope in the water strung across a channel of fast flow surrounded by adjacent slower flows. The switchback in the rope forms where the flow draws out the rope more quickly than the adjacent flow (as illustrated in the bottom panel of Figure 2).
The Sub-Parker and Super-Parker Spirals behave as opposites. The Sub-Parker Spiral has magnetic field lines that are increasingly stretched out, whereas the Super-Parker Spiral has magnetic field lines that contract and then bend back on themselves.
The one-sided, co-rotation-directed tangential flows observed by Parker Solar Probe have been one of the most important and baffling pieces of the puzzle. As shown in Figure 2, differential motion determines the orientation of tangential magnetic field variations, and the field-aligned flow below the Alfven critical point. Tangential flow is oriented in the direction of co-rotation, naturally explaining the observed one-sided tangential flows. The field-aligned flow below the Alfven critical point also causes the development of compressions, and explains the anti-correlation between density and magnetic field strength.
The Alfvenic correlation between velocity and field variations results from the development of Alfvenic structures in the solar wind. Beyond the Alfven critical point, large-scale variations in the flow inevitably develop Alfvenic characteristics. Moreover, the ejection of magnetic field variations close to the Sun are consistent with Alfvenic structures (Schwadron & McComas 2003), as are the exhausts from magnetic reconnection. Therefore, whether the source involves Alfven waves or interchange reconnection exhausts, an outcome is the development of Alfvenic variations with correlation between velocity and field deviations.
Schwadron and McComas (2021) have examined rarefaction regions observed by Parker Solar Probe and find they that are consistent with the Sub-Parker Structure. The observations from Parker Solar Probe confirm the paucity of switchbacks within rarefaction regions. Immediately outside these rarefaction regions, numerous intervals of switchbacks in the magnetic field are observed that are directly associated with abrupt transients in solar wind speed. In contrast to the smooth monotonic speed transition within rarefaction regions, the switchbacks occur in “bursts”. The clustering and plenitude of these bursts occurring in close proximity are consistent with Parker Solar Probe being magnetically connected to the leading edge of the coronal hole. The observations (see Figure 3) confirm the features of the Sub-Parker Spiral and the Super-Parker Spiral: the magnetic field strength, the radial component of the magnetic field, the speed gradients, tangential flows, radial Alfven speed, and the ratio of the sound speed to the radial Alfven speed from the SWEAP and FIELDS observations all conform to predictions based on the Sub-Parker and Super-Parker Spiral within rarefaction regions and solar wind speed enhancements (spikes or jets), respectively. Critically, the predictions associated with the Super- Parker Spiral naturally explain the observations of switchbacks being associated with unexpectedly large and one-sided tangential flows.
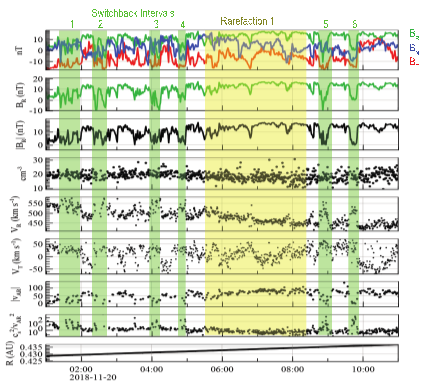
Figure 3. Observations from PSP: FIELDS data (panels 1-3), and SWEAP data (panels 4,5) on November 20, 2018. In panels 6, and 7 we form the radial Alfven speed and the ratio of the sound speed to the radial Alfven speed from the SWEAP and FIELDS observations. Radial distance from the Sun is shown in the bottom panel. Green shaded regions show intervals where switchbacks are observed. The yellow shaded region shows a rarefaction region. From Schwadron and McComas, 2021.
Schwadron and McComas (2021) reveal the origin for switch-backed magnetic field structures and one-sided tangential flows from the formation of the Super-Parker Spiral, the counterpart of the Sub-Parker Spiral. These magnetic structures are produced fundamentally through footpoint motion caused by differential motion and interchange reconnection across regions with a strong gradient in solar wind speed. The existence of these magnetic field structures represents a significant departure from the standard Parker Spiral, and naturally explains fundamental relationships between their solar wind source at the Sun and the magnetic and flow structures out in the heliosphere.
References:
Bale, S. D., et al. 2019, Nature https://doi.org/10.1038/s41586-019-1818-7
Bale, S. D., et al. 2016, Space Science Reviews, https://doi.org/10.1007/s11214-016-0244-5
Balogh, A. et al. 1999, Geophys. Res. Lett., https://doi.org/10.1029/1999GL900061
Borovsky, J. E. 2016, JGR: Space Physics, https://doi.org/10.1002/2016JA022686
de Wit, T. D. et al. 2020, The Astrophysical Journal Supplement Series, https://doi.org/10.3847/1538-4365/ab5853
Fox, N. J., et al. 2016, Space Science Reviews, https://doi.org/10.1007/s11214-015-0211-6
Goelzer, M. L., et al. 2014, JGR: Space Physics, https://doi.org/10.1002/2013JA019420
Gosling, J. & Skoug, R. 2002, JGR: Space Physics, https://doi.org/10.1029/2002JA009434
Gosling, J. T., et al. 2009, The Astrophysical Journal, https://doi.org/10.1088/0004-637X/695/2/L213
Gosling, J. T., et al. 2011, The Astrophysical Journal, https://doi.org/10.1088/2041-8205/737/2/L35
Horbury, T. S., et al. 2018, Monthly Notices of the Royal Astronomical Society, https://doi.org/10.1093/mnras/sty953
Horbury, T. S., et al. 2020, The Astrophysical Journal Supplement Series, https://doi.org/10.3847/1538-4365/ab5b15
Kahler, S. W., et al. 1996, JGR: Space Physics, https://doi.org/10.1029/96JA02232
Kasper, J. C., et al. 2016, Space Science Reviews, https://doi.org/10.1007/s11214-015-0206-3
Kasper, J. C., et al. 2019, Nature, https://doi.org/10.1038/s41586-019-1813-z
Katsikas, V., et al. 2010, Advances in Space Research, https://doi.org/10.1016/j.asr.2010.05.003
McComas, D. J., et al. 2016, Space Sci. Rev., https://doi.org/10.1007/s11214-014-0059-1
McComas, D. J., et al. 2007, Reviews of Geophysics, https://doi.org/10.1029/2006RG000195
Michel, F. C. 1967, Journal of Geophysical Research, https://doi.org/10.1029/JZ072i007p01917
Mozer, F. S., et al. 2020, The Astrophysical Journal Supplement Series, https://doi.org/10.3847/1538-4365/ab7196
Murphy, N., et al. 2002, Geophys. Res. Lett., https://doi.org/10.1029/2002GL015164
Rouillard, A. P., et al. 2020, The Astrophysical Journal Supplement Series, https://doi.org/10.3847/1538-4365/ab579a
Schwadron, N. A., and D. J. McComas, 2021, Astophys. J., https://doi.org/10.3847/1538-4357/abd4e6
Schwadron, N. A. 2002, Geophys. Res. Lett., https://doi.org/10.1029/2002GL015028
Schwadron, N. A., et al. 2020, Astron. Astrophys., https://doi.org/10.1051/0004-6361/202039352
Schwadron, N. A. & McComas, D. J. 2003, Advances in Space Research, https://doi.org/10.1016/S0273-1177(03)00337-5
Schwadron, N. A., et al. 2005, JGR: Space Physics, https://doi.org/10.1029/2004JA010896
Tenerani, A., et al. 2020, The Astrophysical Journal Supplement Series, https://doi.org/10.3847/1538-4365/ab53e1
Weber, E. J. & Davis, Jr., L. 1967, Astrophys. J., https://doi.org/10.1086/149138
Yamauchi, Y., et al. 2004, JGR: Space Physics, https://doi.org/10.1029/2003JA010274