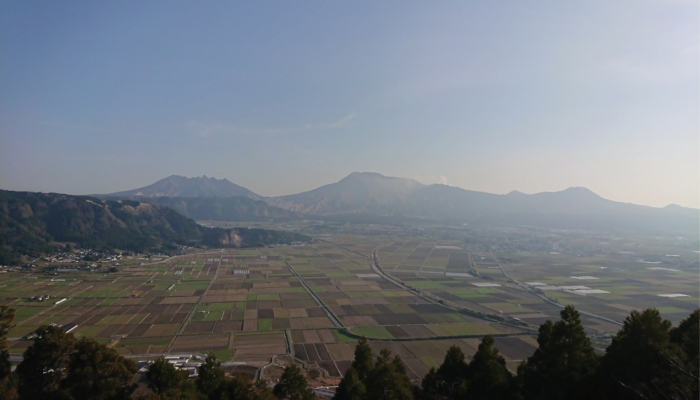
Caldera-forming eruptions represent some of the most dangerous natural hazards we have to face on Earth. If happening today, a single caldera-forming eruption would have a devastating impact on the population living around the volcano, destroy the surrounding environment, and also strongly influence the global climate. But what actually is a caldera and how do scientists interpret their eruptive activity? And are there any signs that can help us to forecast upcoming caldera eruptions?!
A caldera is a wide topographic depression formed by the roof collapse of a shallow magma reservoir, which was (partly) emptied during a ginormous volcanic eruption. Those eruptions can discharge magma volumes from <1 km3 up to enormous volumes of >5000 km3 (e.g. Fish Canyon Tuff at La Garita caldera, USA) producing depressions of up to 40 x 75 km in the regional topography (Branney & Acocella, 2015, Cole et al., 2005). Magmas erupted during caldera-forming events can range in compositions from basalt to rhyolite. Basaltic calderas, however, are usually formed by voluminous effusive eruptions, while silicic calderas (andesitic-dacitic, rhyolitic and peralkaline) are the result of large explosive events (in the following I will only focus on silicic calderas).
In many cases, several caldera-forming events occur at a such volcanic system in the course of several 100.000s of years (e.g. Aso caldera, Japan experienced 4 caldera-forming eruptions between 266 and 86 ka BP). But also many calderas experienced only one caldera-forming event (e.g. Long Valley caldera, USA was formed by the voluminous Bishop Tuff eruption). However, scientists found comparable activity periods characterized by distinct evolutionary paths in most caldera systems, suggesting cyclic volcanic activity at such systems (Smith & Bailey, 1968; Lipman, 1984; Cole et al., 2005; Branney & Acocella, 2015; Forni et al., 2018; Bouvet de Maisonneuve et al., 2021). This activity can be summarized as followed:
1. A period of pre-collapse activity
2. The catastrophic caldera formation
3. Post-collapse recovery or the possible cessation of the system.
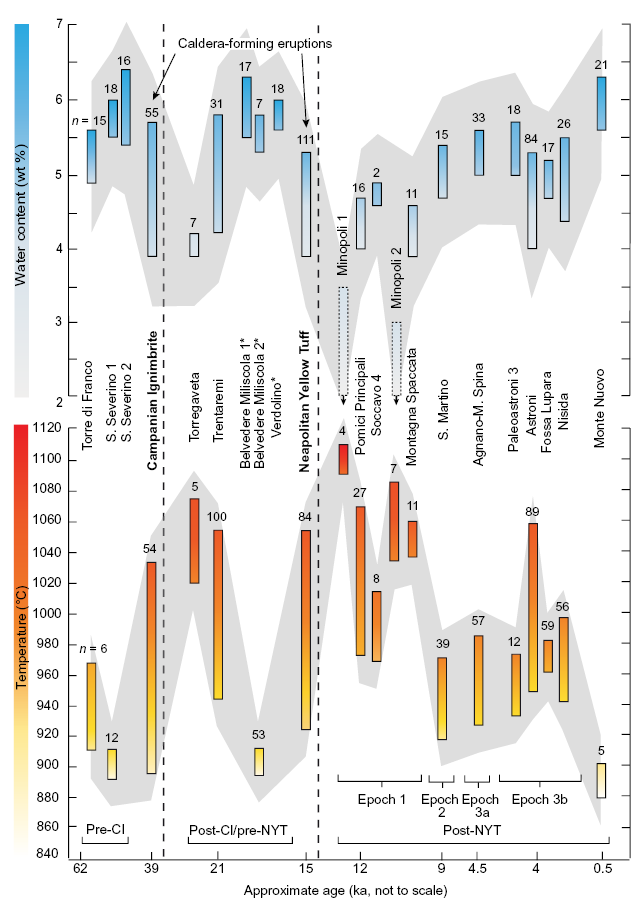
Time paths of equilibrium temperature and water content in several Campi Flegrei units (figure from Forni et al., 2018).
Typically, each period of the caldera cycle is characterized by distinct variations in the composition and physical properties of the erupted magmas, the eruption frequency, the spatial distribution and size of eruptions, and the architecture of the underlying magmatic system. The pre-collapse activity leads to the thermal maturation of the upper crust by incremental accumulation of silicic magmas in the shallow magma reservoir. This process is accompanied by a few eruptions of small volumes of differentiated material, separated by long dormancy intervals. The pre-collapse state then culminates in the caldera-forming eruption. Forni et al., 2018 observed that prior to the climactic caldera formation, a gradual decrease in temperature and an increase in the dissolved water content might be signs of an upcoming caldera-forming eruption. After the caldera-forming event, the post-collapse recovery period is entered. This period is characterized by more frequent but small volume eruptions of less differentiated material as new recharge enters the emptied and mostly crystallized upper crustal reservoir. From here the system now can shift again into the pre-collapse state starting a new caldera cycle or activity might subside leading to the cessation of the cycle.
Bouvet de Maisonneuve et al., 2021 reworked this prevailing model recently, in order to include more details on the temporal evolution of the state and properties of the subvolcanic system. They subdivided the caldera cycle in the following five stages:
1. Incubation: thermal maturation of the upper crust
The incubation period is characterized by the presence of a network of small sills and dikes with only a few to no associated long-lived storage zones of evolved magma in the upper crust. Usually magmatism in this stage is dominated by mafic to intermediate small, but frequent eruptions.
2. Maturation: magma growth & differentiation
The maturation period typically shows an initially high but over time decreasing eruption frequency of intermediate to silicic material. This results from the development of a single or multiple magma reservoirs in the upper crust. Over time those reservoirs grow in size accompanied by an increase of the degree of differentiation and volatile content. The build-up of an extensive mush is suggested to take place in this period.
3. Fermentation: extensive development of an exsolved volatile phase
In this stage of the caldera cycles a continuously present volatile phase exsolves in one or more long-lived magma reservoirs that already formed in the upper crust. This period is hence characterized by runaway growth of the magma reservoir/s leading to infrequent eruptions of very evolved material accompanied with large scale volcanic unrest like deformation, the occurrence of seismic swarms or increasing hydrothermal activity. This phase then culminates in the caldera-forming eruption.
4. Recovery: active recharging of the shallow reservoir
The recovery stage follows the climactic caldera formation. This means that the reservoir was largely emptied so that no silicic material or exsolved volatiles are present in the reservoir anymore. Mafic to intermediate recharge that enters the reservoir now dominates the shallow reservoir delivering new heat and material, which might melt remnants of the crystal mush left behind in the reservoir. This results in the eruption of less homogeneous material including less evolved recharge magmas and leftover material from the caldera-forming eruption, which might carry signs of cumulate rejuvenation.
5. Death of the caldera cycle
Concomitant with waning recharge fluxes the upper crustal reservoir cannot recover from the caldera forming eruption leading to the death of the current caldera cycle. This phase is characterized by a low/ average eruption frequency, where plutonic trapping of magmas dominates.
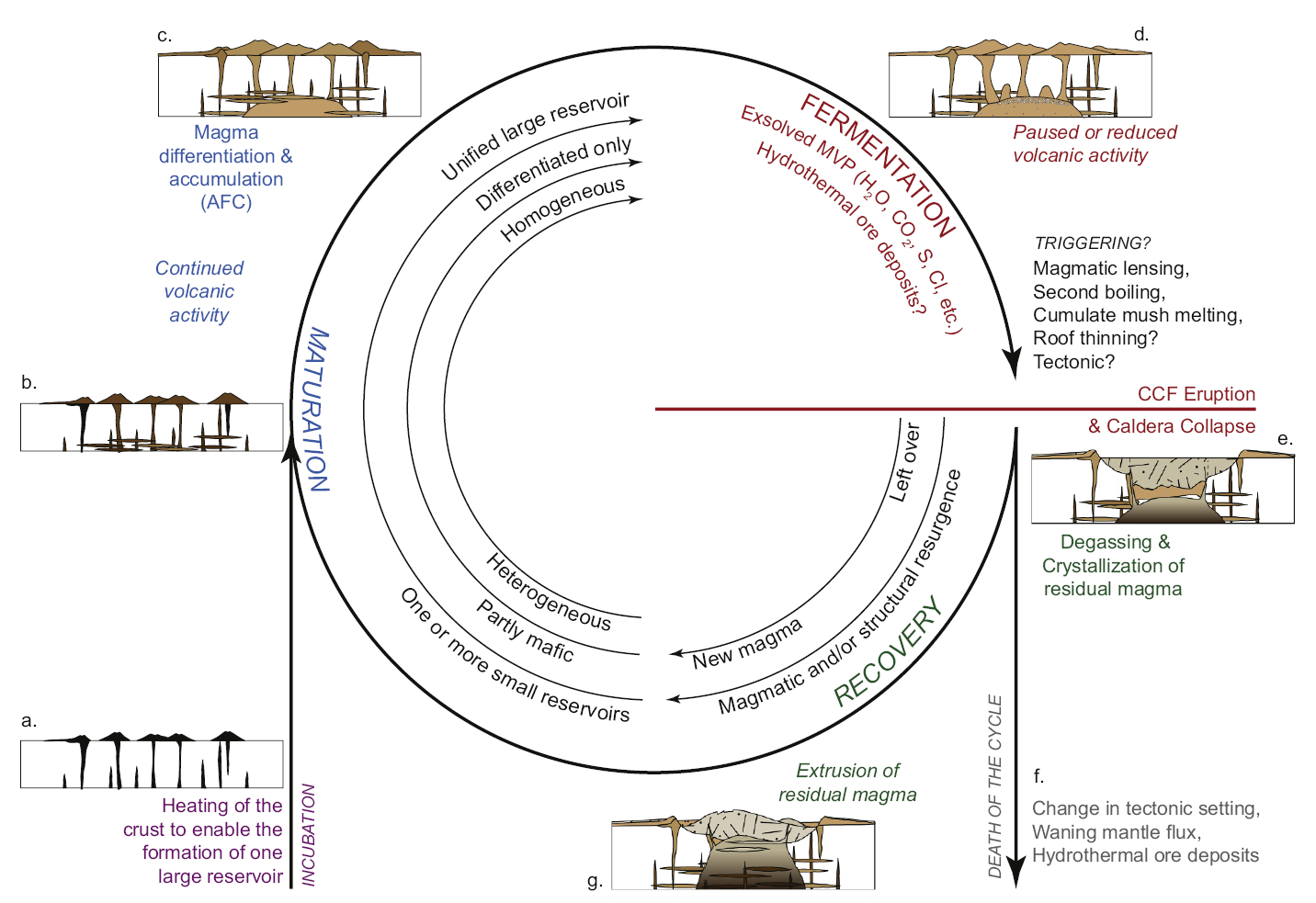
Schematic representation of a caldera cycle after Bouvet de Maisonneuve et al., 2021 (from Bouvet de Maisonneuve et al., 2021).
This refined model of caldera cycles helps scientists to better understand whether the currently observed activity of an active caldera system is potentially leading towards another caldera-forming event and hence requires improved volcano monitoring, or if the system is in the recovery stage and might need another 10.000s to 100.000s of years until another caldera formation could occur. However, many questions such as “When is the transition from recovery to maturation stage?” or “How to interpret volcanic unrest at active caldera systems?” still remain open and limit our abilities to precisely forecast those extremely hazardous eruptions.
References: Branney, M., & Acocella, V. (2015). Calderas. In The encyclopedia of volcanoes (pp. 299-315). Academic Press. Cole, J. W., Milner, D. M., & Spinks, K. D. (2005). Calderas and caldera structures: a review. Earth-Science Reviews, 69(1-2), 1-26. de Maisonneuve, C. B., Forni, F., & Bachmann, O. (2021). Magma reservoir evolution during the build up to and recovery from caldera-forming eruptions–A generalizable model?. Earth-Science Reviews, 103684. Forni, F., Degruyter, W., Bachmann, O., De Astis, G., & Mollo, S. (2018). Long-term magmatic evolution reveals the beginning of a new caldera cycle at Campi Flegrei. Science advances, 4(11), eaat9401. Lipman, P. W. (1984). The roots of ash flow calderas in western North America: windows into the tops of granitic batholiths. Journal of Geophysical Research: Solid Earth, 89(B10), 8801-8841. Smith, R. L., & Bailey, R. A. (1968). Resurgent cauldrons. Memoir of the Geological Society of America, 116, 613-662.
Ali Jaffri
This was a very useful summary! Thank you Franziska!