What comes to your mind when someone says ‘theropod dinosaurs’ to you? Does the mind conjour images of Tyrannosaurus rex noshing on lawyers, packs of voracious Deinonychus ambushing unsuspecting ornithopods, or perhaps you’re quite progressive and thought about flocks of birds migrating south for the winter. Sticking strictly to the Mesozoic (before 65.5 million years ago), most people probably think of hungry flesh-tearing beasties as the archetypal theropods. There were, however, 3 groups of theropods that were herbivorous! These groups are relative newcomers to the world of palaeontology, many having been recently discovered in China, or trophically reclassified as new information on diets were uncovered.
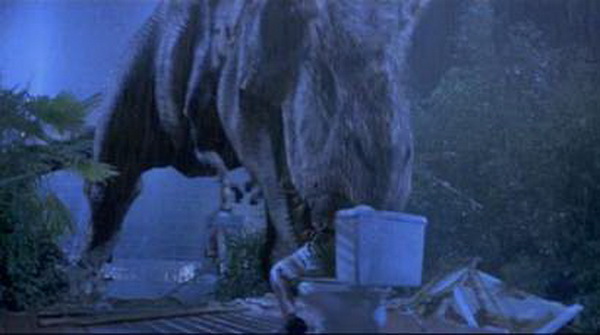
Nom
Herbivory is a rather odd adaptation. There are a suite of physiological and morphological characteristics required for the ingestion and digestion of high-fibre forage. For example, modern cows (and possibly some groups of dinosaur such as the sauropods) have a symbiotic gut flora that helps to break down the complex cellulose in plants, and hadrosaur dinosaurs had the most complex tooth system known in evolutionary history.
Herbivores with a higher body mass are strategically adapted to increase digestive efficiency. This occurs in many extant herbivorous clades (e.g., ruminants, elephants, people who only eat McDonald’s ‘salads’). This is due to a relationship known as ‘gigantothermy’, whereby a larger body size decreases the surface-area to volume ratio, permitting a higher body temperature with a relatively lower energy expenditure (assuming that metabolic rates remain constant), something pretty beneficial to animals. As such, the selective advantage of increasing body size has been proposed for many groups, including Palaeogene and modern mammals and non-avian dinosaurs.
A new study has tested this relationship in herbivorous coelurosaurian theropods, namely members of Ornithomimosauria, Therizinosauria, and Oviraptorosauria. Currently, despite dinosaurs generally being acknowledged as the biggest terrestrial animals ever, the picture of their body size evolution is rather complicated. Some groups, such as ornithiscians, show a general trend of increasing body mass through time, others towards miniaturisation (avian-line theropods), or stasis (early dinosaurs). The role of herbivory within these patterns is still largely a mystery, especially when viewed within the larger system of biological, ecological, and environmental parameters that can potentially influence body sizes. New key fossils of the three coelurosaurian subclades, however, have allowed palaeontologists to paint a more clear picture of these key evolutionary and dietary transitions. These groups are intriguing to test the correlation of herbivory and body size on, due to the fact that in each group, calculated body masses imply that relative gigantism occurred in members of each (greater than 3000kg!)
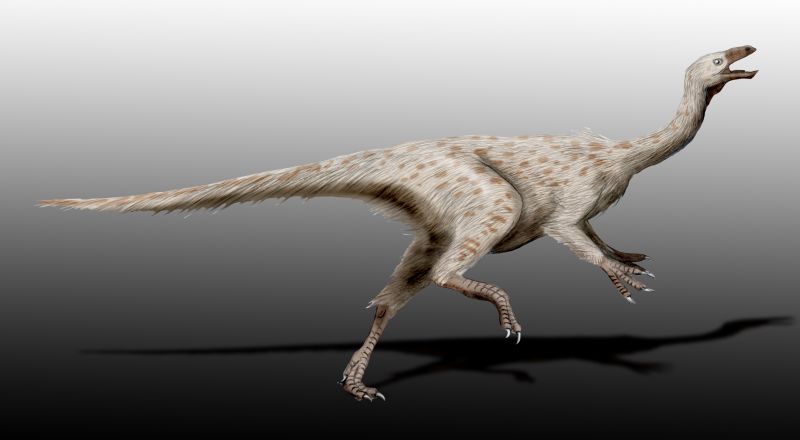
Reconstruction of Beishanlong grandis (by Nobu Tamura)
Methods
Estimating the mass of extinct organisms is a pretty tough job. Many dinosaur species we know of are not known from complete skeletons. In fact, many are only known from one or two bones, or small collections of disarticulated bits and bobs. The more complete the skeleton, the rarer they are, as a general trend. This makes mass estimation exceedingly problematic, as you have to rely a lot on extrapolation using various proxies, in this case, the length of the femur, to get a rough estimate of the mass of the whole skeleton, as well as the small matter of all the fleshy parts. Deriving relationships between long bone measurements and body sizes or masses in extant organisms goes some way to acting as a guide, but really the best we have using these simple methods is an educated guess. Such methods have recently come under scrutiny too, but fortunately other methods do exist to estimate body masses [see this paper and this one too, both free!].
The real statistical wizardry comes next. To analyse body mass in a phylogenetic context (based on evolutionary relationships), the authors employed a method known as phylogenetic generalised least-squares, which takes the lengths of the branches between taxa, based on the chronostratigraphic distance they represent. Body mass estimates were constructed using a maximum likelihood approach under different evolutionary models (e.g., Brownian Motion), and fitted to phylogenetic trees for the three subclades. The different evolutionary models were assessed using a criterion known as Akaike weighting, which is a method of calculating how well different evolutionary models fit the data in a probabilistic environment. The authors also conducted numerous sensitivity analyses on the modelled data, which are methods of testing how well the analysis holds up when it is perturbed through, for example, sub-sampling techniques. I’ll be doing similar things during my PhD, so hopefully will be able to convey these methods a little better once I’ve conducted them first hand!
What did they find?
In macroevolution, there are certain trends that are recurrent through time and space. One of these is the latitudinal biodiversity gradient, whereby biodiversity tends to increase towards the equator, a pattern that is prevalent in most modern groups. Another is Cope’s Rule, where size increases within individual groups resulting from directional evolution. In all three coelurosaurian subclades, each independently contains members that attain body sizes greater than 6000kg (as big as the biggest tyrannosaurs!) in the Maastrichtian period (the end of the Cretaceous, at the end of which all non-avian dinosaurs went extinct). However, the evolutionary models used suggest that this does not result from directional selection, providing evidence against Cope’s Rule, consistent with several recent studies on extinct and extant organisms (it’s hardly a ‘rule’ any more..). As different-sized members of each clade are from different time periods and different environments, it suggests that there is some sort of taphonomic or habitat-sampling bias confounding the true pattern of body mass evolution.
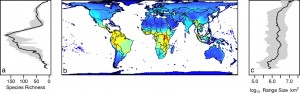
The latitudinal biodiversity gradient in modern mammals (click for larger; source)
A recent study (conducted by Roland Sookias, as part of his MSc thesis on the same course as me!) suggested that passive processes drove body size evolution in three groups of herbivorous archosaurs (Sauropodomorpha, Ornithischia, and Aetosauria), which coupled with this new analysis strongly indicates that herbivory was not a driver of body size evolution in archosaurs. What this demonstrates is that evolutionary patterns are not simple, not in the slightest, and in fact are likely to be the results of the interplay between numerous ecological, physiological, and environmental parameters. The alternative, and slightly more depressing possibility, is that the fossil record is just too naff to readily preserve these important evolutionary and ecological patterns and processes. Furthermore, we might not be looking at the right aspects of herbivory – this diet, as mentioned, requires many specific morphological and physiological adaptations, any one of which, or any combination of which, may contribute towards the evolutionary patterns of body size we see preserved in the fossil record (something I’m working on a bit extending from my MSc project).
So yeah, there you have it – there is little evidence that herbivorous theropod dinosaurs show a pattern of body mass change relating to herbivory. However, what did drive the evident patterns of increasing body mass through time is still unresolved. Final point: will the fossil record ever be good enough so that we can actually answer these types of questions..?