This post is kind of a continuation of Laura Roberts excellent guest post on the Solar Storms and the Earth’s Magnetic field. However, this is a bit of a different spin on it. I am not writing about what get’s kept out, but rather what slips by the shield and gets in. Of course, I am speaking about cosmic rays and the wonderfully useful isotopes they produce that rain down upon us. Yes, it is literally raining isotopes…all the time! I know that this sounds weird, when I first learned about this phenomenon it came as a complete surprise to me. Not only are isotopes raining down all the time, but there is a lot that we can learn about our planet by analyzing these amazing by-products of cosmic ray collisions.
What are cosmic rays?
Cosmic rays are incredible things. The Earth is constantly being bombarded by them and we are protected from this massive influx of extra-terrestrial radiation by our magnetic field and atmosphere, which deflects about 90% of the low energy cosmic rays. Check out last weeks awesome guest post about Earth’s magnetic field. There are two types of cosmic rays. Primary cosmic rays generally originate outside of our solar system and travel throughout space occasionally bumping into things like planets. In fact, it has only recently been discovered exactly where they come from. In February, 2013 a paper came out in Science called: Evidence Shows That Cosmic Rays Come From Exploding Stars that (surprise) stated primary cosmic rays are produced by exploding stars aka. supernovae in which an ancient star blows up, releasing massive amounts of energy, elements and cosmic rays!

Kepler’s Supernova (Source)
Primary cosmic rays are 99% protons and alpha particles (two protons and two neutrons) and the remaining 1% are the nuclei of heavy atoms or beta particles (electrons). These bits of atoms and radiation fly around in space and bang into everything. Sometimes, actually more often than sometimes, they bang into the Earth. In fact, they bang into the Earth a lot! There are billions of collisions every day. Some of the particles crashing into the Earth have low energies while others have high energies. The frequency that these low energy particles collide is many times greater than high energy ones. There are about 10,000 collisions per square meter per second for giga-eletronvolt particles. For the higher energy rays the rate of collision is less. They might arrive with a frequency of 1 per square metre per second, as is the case with tera-electronvolt particles. The decrease in collision frequency continues as energy increases up until the very highest energy of the exa-electronvolt which collides about 0nce per square kilometre per century.
When cosmic rays pass through the Earth’s magnetic field is when things start to get really interesting, from a geochemical perspective that is. When primary cosmic rays enter the Earth’s atmosphere they start banging into all of the molecules and atoms floating around up there and produce secondary cosmic rays. Basically imagine a bowler throwing a strike. The bowling ball represents the primary cosmic ray and the pins are the molecules in the atmosphere. When the ball collides with the pins they fly off in every direction, which is analogous to the process occurring constantly in our atmosphere. When this takes place in the atmosphere the collision is so energetic the molecules and atoms can actually break apart. This is called spallation and results in a cascade of neutrons, protons and atoms called an air shower.
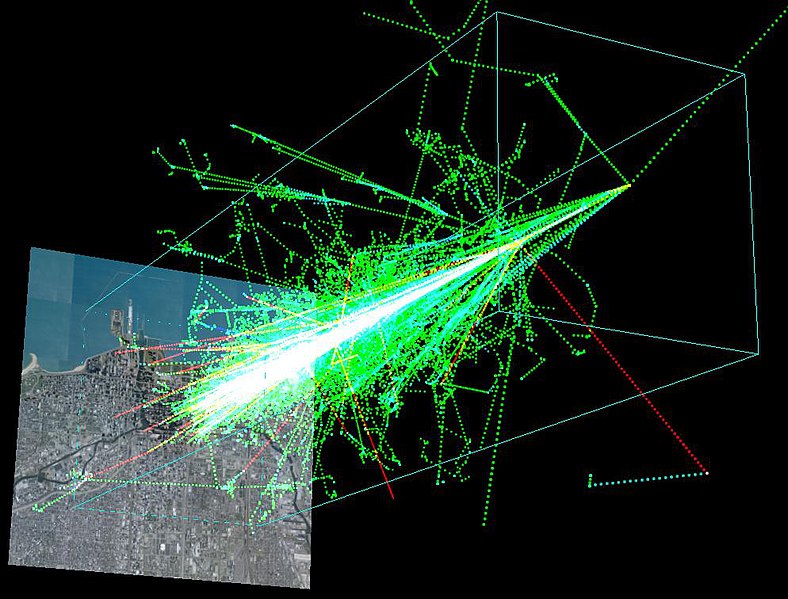
A simulation of a cosmic ray shower formed when a proton with 1TeV (1e^12 eV) of energy hits the atmosphere about 20km above the ground. The ground shown here is a 8km x 8km map of Chicago’s lakefront. This visualization was made by Dinoj Surendran, Mark SubbaRao, and Randy Landsberg of the COSMUS group at the University of Chicago, with the help of physicists at the Kavli Institute for Cosmological Physics and the Pierre Auger Observatory. (Source)
What is produced?
Air showers produce all sort of radioactive isotopes that are formed no other way. Some are very short lived and decay away quickly, while others are extremely long lived and have half lives of millions of years, allowing them to accumulate in the environment.

A list of the major cosmic ray isotopes and their half lives. The ones in bold have geologic applications.
Other isotopes are produced when cosmic rays reach the ground and collide with minerals. This can cause other isotopes to form within the mineral crystal lattice. For example, the cosmogenic isotope, aluminum-26 is produced when a cosmic rays strikes a quartz crystal and transforms some of the silicon in the quartz structure into aluminum. These are referred to as in-situ cosmogenic isotopes.
The next part of the process concerns what happens to all of these isotopes once they have sprung into existence thousands of metres above our heads. The fate of these isotopes is varied and they all live in the atmosphere for a little while before becoming part of the hydrologic cycle. Eventually they fall down to Earth either stuck onto aerosols or incorporated into rain and begin to interact with water bodies, enter groundwater, stick to soil particles or get absorbed into people, plants or animals. Essentially, they begin cycling through the environment until they decay away. Since these isotopes get cycled through various parts of the environment we can use them as tracking tools to trace the pathways they take and learn about their interactions with other components of environmental cycles.
What is the use?
Cosmic rays isotopes are useful for more than just getting a good tan! In fact, the list of possible questions that can be applied to cosmogenic isotopes just goes on and on. I’ll just briefly cover a few of them since each one of these applications is a whole blog post by itself I’ll just give the highlights here.
Exposure age dating: Exposure age dating is one of the principle uses of cosmogenic isotopes and several different ones have been employed to this end. Exposure age dating is when we use cosmogenic isotopes to date how long something has been exposed to secondary cosmic rays at the Earth’s surface. For example, a rock at the toe of a glacier may have just been exposed to cosmic rays a year ago and its exposure age date will tell us this. Another rock, 20 metres further from the glacier toe may have been exposed 5 years ago and this difference will be recorded. Basically, the clock starts ticking once cosmic rays are able to reach the rock or bone, or whatever material is being dated. Exposure age dating has the power to date materials that range from only a few thousand years to millions of years depending on the half lives and concentrations of the nuclides in question. The way exposure dating works is that when a secondary cosmic ray impacts a rock containing Si (silicon) or O (oxygen) some of the neutrons on the silicon or oxygen are knocked off and the result is the production of aluminum-26 or beryllium-10 right in the mineral lattice. One common mineral that contains both silicon and oxygen is quartz. Quartz is pretty much everywhere and in everything, which opens the door for a wide range of materials that can be exposure age dated. All they have to contain is a bit of quartz. The decay of 26Al and 10Be allows the material to be dated. There are other isotopes that can be used for exposure age dating besides these two and other minerals that contain Si or O can also be used as well. The figure below shows the wide range of applications that exposure age dating can be applied to. For example, if you want to date a volcanic eruption it is easy to exposure age date the lava or ash, both of which have a lot of Si. It is also possible to date landslides, erosion rates, burial ages, the list goes on and on. There are many other isotopes that can be used as well besides 26Al and 10Be.

Figure showing all of the different applications of exposure age dating. Ivy-Ochs, S., & Kober, F. (2008). Surface exposure dating with cosmogenic nuclides. Quaternary Science Journal, 57(1-2), 179–209.
CLOUD Project: The CLOUD project is a CERN experiment that is investigating the possible linkage between cosmic rays and cloud formation. It has been hypothesized that cosmic rays can cause clouds to form by forming aerosols and it has been noticed by satellites that an increase in the cosmic ray bombardment can increase the amount of cloud cover. Cloud cover has a profound influence on the Earth’s energy balance and hence climate. It has been proposed that throughout geologic time changes in the cosmic ray flux have been responsible for climate change. However, proving this is difficult given the length of time in question and the evolving states of the cosmic ray flux and Earth’s magnetic field. However, CLOUD has set out to find the linkage between cosmic rays, clouds and climate if one exists.
Carbon-14: Carbon 14 is unquestionably the most used of any of the cosmogenic isotopes. It has applications in geology, archaeology, paleontology, anthropology, hydrogeology, and many other -ologies as well. 14C is produced primarily when neutrons collide with nitrogen in the atmosphere replacing a proton in the nitrogen nucleus and transforming it into carbon-14, although atmospheric nuclear weapons testing also produced a substantial amount of 14C. The primary use of 14C, or radiocarbon, is radiocarbon dating. Once radiocarbon is produced it enters the global carbon cycle and disperses throughout the environment. Since it is part of the carbon cycle it becomes incorporated into all living things, including people and animals. 14C continues to enter our bodies while we are alive. Once we die, there is no further addition of 14C and therefore, the clock starts ticking on its use as a dating tool. 14C has a 5,730 year half life and is therefore useful as a dating tool for biological materials up to ~45,000 years ago.
Cosmic rays are some of the most mysterious, yet useful phenomena on our planet and have allowed for incredible advances in many sciences. As new, more sensitive technology becomes available look for the use of cosmic rays and the isotopes they produce to accelerate as we find new questions they can be used to answer.
Cheers and thanks for reading,
Matt