As I touched upon in our first post, we can use the magnetic properties of minerals in sediments (and other environmental materials) to understand changes in environmental and climatic conditions. This is known as environmental magnetism. The basic idea is to identify links between the magnetic properties of a material and environmental conditions and depositional processes. This approach is not as modern as you might think and was first used back in 1926! Understanding and characterising how and in what quantities magnetic minerals form can give key indicators of past climates. A great example of how useful this tool can be for understanding rates of deposition, geochemical conditions and past climate changes was shown in our post last week. Magnetic iron oxides and sulphides can form and be dissolved in deep-sea sediments, depending on the geochemical conditions and this can be used to identify ghost sapropels, (Langereis & Dekkers, 1999). Combining evidence for change in the magnetic signature of sediments and changes in composition can indicate changes in the climatic or tectonic setting in which the material was being deposited.
Magnetostratigraphy as a dating tool
In addition, magnetostratigraphy can be used to date sedimentary (and volcanic) sequences. It is, essentially, a correlation technique, which aided by independent isotopic ages, can be used to date a sedimentary section or core. The Global Magnetic Polarity Time Scale (GMPTS) is used for this . The direction of the field recorded in a stratum can be normal or reversed and this will coincide with a known normal or reversed chron of a given age. In the 1950s the first GMPTS, of sorts, was established using the sea floor magnetic anomaly patterns (the familiar bar code type outline seen spreading away from the North Atlantic Ridge). However, it was later on, when each reversal was accurately dated using the astronomical polarity time scale (APTS ) that magnetostratigraphic became a valuable chronology tool. The rates of sediment accumulation within a sequence or core can also be established by plotting the age of the each reversal versus the stratigraphic level at which the reversal is found, giving deposition rates in meters per million years.
What triggered sapropel formation?
We explained in our last post some of the proxy techniques capable of distinguishing sapropels from the background sediment matrix. A dramatic environmental change must have been necessary to create the depositional conditions suitable for their formation. Investigating the nature of this change has been a key task for palaeoceanographers over several decades!
The critical condition for sapropel formation was deep water anoxia (water depleted in oxygen) within the eastern Mediterranean Sea. So, periodically, dissolved oxygen was unable to reach the deeper sea because the water column was vertically stratified; in other words, surface water had quite low salinity while the deeper waters were highly saline (Rossignol-Strick et al. 1982). An influx of freshwater to the Mediterranean would have decreased the salinity of its surface waters; scientists have therefore posed the questions “from where did this increased flow of freshwater come and what was its trigger?” A number of hypotheses have been put forward over recent decades, all of which invoke a strong link between climate and sapropel formation, although the primary trigger has been more widely debated.
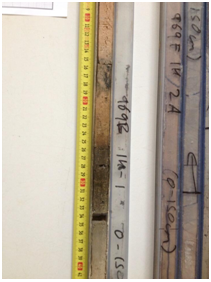
The darker sapropelic layer is clearly visible in this photographic core log. Photograph used with the kind permission of Dr Mike Rogerson, University of Hull.
The original hypothesis from E. Olausson (1961) proposed massive volumes of meltwater from Eurasian ice-sheets entered the Mediterranean from the north at the beginning of warm interglacials. While this has undoubtedly occurred periodically through the Quaternary, improved dating (using magnetostratigraphy as described above, for example) indicates a mismatch between the timing of sapropel formation and meltwater influx.
More recent research linked the formation of sapropels to enhanced solar insolation. Insolation refers to the amount of solar radiation reaching an area of the Earth’s surface, which varies through the day, annually and on longer timescales (i.e., Milankovitch cycles). Over these longer timescales, it appears phases of insolation maxima during the Northern Hemispheric summer caused stronger monsoons to form over northern Africa, bringing more intense rainfall. As a result, flow in the River Nile (and likely in other rivers draining into the Mediterranean from North Africa that have since dried up) was greatly increased, delivering substantial volumes of freshwater.
The most widely accepted hypothesis today expands on the Nile freshwater hypothesis and suggests warmer sea surface temperatures (due to higher insolation) occurred simultaneously (Emais et al. 2003). Together, the influx of freshwater and warmer sea surface temperatures were sufficient to create an upper water layer of sufficiently low density to interrupt circulation and create the oxygen-poor conditions at the sea bottom necessary for the formation of sapropels.
Olausson, E. (1961) Studies in deep-sea cores. Reports of the Swedish Deep-Sea Expedition, 1947-1948, v.8, Sediment cores from the Mediterranean Sea and Black Sea. [d1]