Since 1998 the American Geosciences Institute has been running an annual celebration of all things geoscience – Earth Science Week. From its inception, Earth Science Week has grown in popularity and is now celebrated across the planet. This year Earth Science Week will run from 11 to 17 October and the theme of the week is “Earth Materials in Our Lives.” As the American Geoscien ...[Read More]
Earth Science Week 2020: Earth Materials in Our Lives – an A-Z!
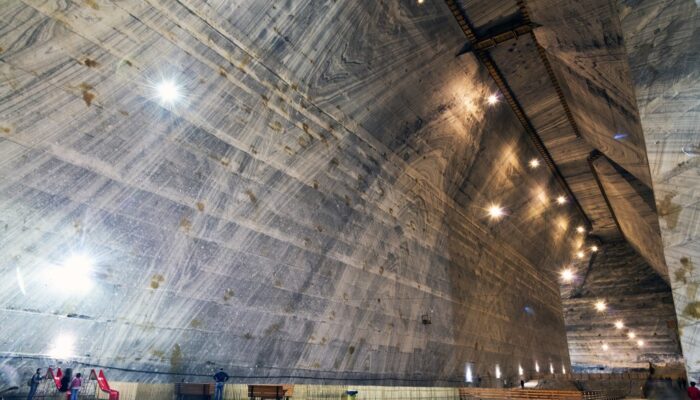