Thirteen years ago, a roughly 251 km2 chunk of ice (or 97 miles2) broke off Greenland’s Petermann Glacier. This Amsterdam-sized piece of ice was the largest to calve in the Arctic since 1962. The massive iceberg traversed the Nares Strait, which lies between Canada’s Ellesmere Island and Greenland, and into the northern part of Baffin Bay—the northwestern-most arm of the Atlantic Ocean, before eve ...[Read More]
A chunk of ice the size of Amsterdam: how the calving of Greenland’s glaciers has changed since the 2010 Petermann Glacier event
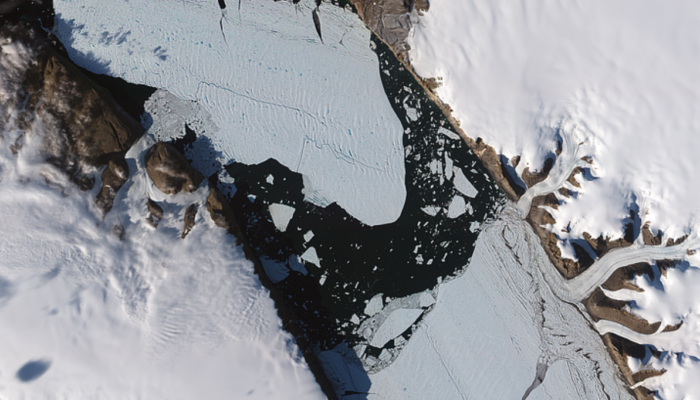