‘What I wish someone told me early in my career’ is a new Geolog series that aims to provide valuable insights and guidance to early-career professionals within the European Geosciences Union (EGU) community. Each month, I will interview a staff member of EGU to share their personal career journey, experiences, challenges faced, and the tips they wish they had received earlier in their ...[Read More]
Tropical rainforest, the lungs of our planet, might be releasing more than just CO2!
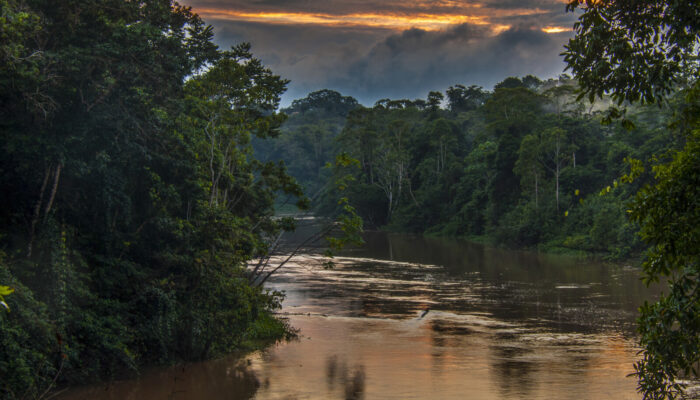
When I was thirteen years old, my family and I almost lost our lives due to a carbon monoxide (CO) leak. I never stopped thinking about that incident even though it happened over twenty years ago. Not only because it was a premature realisation of my own mortality, but also because of how sneaky it was: We did not smell it, see it, hear it, or feel it. It was a subtle and slow killer that could ha ...[Read More]
GeoTalk: meet Morelia Urlaub, researcher of underwater landslides!
Hi Morelia. Thank you for joining us today! Could you tell our readers a bit about yourself and your research? Hi, I am Junior Professor for Marine Geomechanics at GEOMAR Helmholtz Centre for Ocean Research Kiel and Kiel University in Germany. I graduated at the University of Bremen (Germany) and did my PhD in 2013 at the National Oceanography Centre Southampton in the UK. After a short postdoc th ...[Read More]
Why do we keep dismissing drought?
“If you see me, then weep” Like the foreboding inscription witnessed by Dante as he passed through the gates of Hell, the inscription chiselled into the so-called “hunger stone” marks the passing of a threshold into suffering. As the hunger stone emerges from the dwindling waters of the Elbe River, Czechia, it reveals a history of desiccation. Where spiritual torment is pro ...[Read More]